ÁREA
Química Ambiental
Autores
Oliveira, H.L. (UNIVERSIDADE FEDERAL DO RIO GRANDE DO NORTE) ; Batista, M.V.A. (UNIVERSIDADE FEDERAL DO RIO GRANDE DO NORTE) ; Gondim, A.D. (UNIVERSIDADE FEDERAL DO RIO GRANDE DO NORTE) ; Martínez-huitle, C.A. (UNIVERSIDADE FEDERAL DO RIO GRANDE DO NORTE) ; Castro, S.S.L. (UNIVERSIDADE DO ESTADO DO RIO GRANDE DO NORTE) ; dos Santos, E.V. (UNIVERSIDADE FEDERAL DO RIO GRANDE DO NORTE)
RESUMO
This study presents an innovative approach to simultaneously producing green hydrogen and treating cashew nut processing effluent through electrochemical treatment. A two-compartment electrochemical cell with an anion exchange membrane was used to isolate the anode for cashew nut shell liquid treatment and the cathode for hydrogen production. Results showed that higher current density resulted in a higher rate of COD removal, with a removal rate of 53.28% achieved at 100 mAcm-2. In addition, NaOH concentration was found to affect CNSL oxidation, with higher concentrations resulting in decreased COD removal rates. The results also indicated a direct relationship between current density and hydrogen production.
Palavras Chaves
cashew nut shell liquid; electrochemical oxidation; green hydrogen
Introdução
Hydrogen is one of the most abundant elements, found in water and hydrocarbons, and used in various industrial applications such as ammonia and methanol production. However, the current method of obtaining hydrogen from the steam reforming of methane or other hydrocarbons results in significant CO2 emissions. Recently, the possibility of producing green hydrogen (H2) from electrolysis of water using renewable energy sources has been widely studied, offering a promising solution to address climate change challenges (Miller et al., 2020). Hydrogen has been proposed as a versatile alternative to fossil fuels for power generation in the domestic heating, industrial, and transportation sectors. This shift towards a hydrogen-based economy/society has the potential to transform the global energy economy. Despite its potential, the demand for fossil fuels still dominates due to cost-related issues (NOUSSAN et al., 2020). One possible solution is a hybrid approach, where the electrochemical process is used to treat wastewater, with the anode mediating the oxidation of organic compounds, while the cathode produces H2 by water reduction in alkaline media. Taking into account two processes using an anion exchange membrane, for example, effluent can be treated while producing H2 (CARDOZO et al., 2022). The cashew-nut shell liquid (CNSL) can result in highly harmful effluent to the environment, even after conventional treatment during the cashew nut processing. The CNSL is mainly composed of phenolic compounds such as cardol, which can be toxic and carcinogenic. Therefore, efficient treatment methods are needed to ensure proper disposal, and electrochemical treatment can be an effective candidate (MGAYA et al., 2019). With that, the objective of this study was to present a highly sustainable and innovative approach to the production of green hydrogen while treating the effluent of CNSL. The results contribute to face the current challenges of energy and waste management, seeking solutions that minimize environmental impacts in several domains.
Material e métodos
All reagents used in this study were of analytical grade and the solutions were prepared with ultrapure water obtained from a Millipore Milli-Q system with resistivity > 18 MΩ at 35 °C. The supporting electrolyte, NaOH, were supplied by Dinâmica Química LTDA. The CNSL used in this study was obtained from a cashew nut processing industry located in Mossoró, Rio Grande do Norte, Brazil (Medeiros et al., 2020). The electrochemical cell used was divided in two-compartments (anodic and cathodic) separated by an anionic exchange membrane, the anodic compartment has a volume of 0.02 L and the cathodic compartment has a volume of 0.04 L with a gap of 3.4 cm between electrodes. The anodic compartment was operated in flow mode and was connected to a tank containing 1L of CNSL effluent. On the other hand, the cathodic compartment was operated in batch mode and was connected to an inverted burette filled with water, which allowed for the collection of the generated hydrogen and subsequent measurement of the volume produced. To conduct the experiments, boro-doped diamond electrode with an area of 10 cm2 was utilized as the anode, while a 316-type stainless-steel (SS) was used as the cathode. The experiments were carried out at room temperature, with three current densities (j = 40, 70, and 100 mA cm-2) in a supporting electrolyte of NaOH at 1.0 mol L-1, and with a CNSL concentration of 0.01%. Furthermore, the impact of NaOH concentration on the oxidation of CNSL (0.01%) was evaluated by varying the NaOH concentration (0.125, 1.00, and 2.00 mol L-1). At predetermined time intervals, samples were collected for the analysis of chemical oxygen demand (COD) and for determining the volume of hydrogen produced. All experiments were carried out under galvanostatic conditions and were powered by a solar radiation-based energy source (Henrique et al., 2021).
Resultado e discussão
When it comes to electrochemical oxidation, several parameters such as current
density and electrolyte concentration can significantly influence reaction
efficiency. To investigate this relationship, a study was conducted where
current density was varied between 40, 70, and 100 mA cm-2 in 1.0 mol
L-1 of NaOH. As observed in Figure 1a, as current density increases,
there is an increase in DQO removal rate, with the current density of 100 mA
cm-2 showing the highest DQO removal (53.28%) at the end of
electrolysis, followed by 70 mA cm-2 (45.68%) and 40 mA
cm-2 (38.54%). A similar behavior was observed in another study by
Medeiros et al. (2020), who used an open-undivided cell to degrade CNSL.
In their results, increasing current density resulted in a higher DQO removal
rate, achieving results close to 80% for 100 mA cm-2. Current density
is responsible for controlling the flow of electrons in the electrochemical
system, which has a direct implication on the accumulation of electro-generated
oxidative species such as hydroxyl radicals.
To reduce the ohmic resistance and favor CNSL solubilization, NaOH was used as
electrolyte in different concentrations (0.125, 1.0 and 2.0 mol L-1),
keeping the current density fixed at 40 mA cm-2. As seen in Figure
1b, the NaOH concentration that provided the highest COD removal was 1.0 mol
L-1, with a value of 38.54%. The 0.125 mol L-1
concentration showed a similar result, with 35.90% removal, while the 2.0 mol
L-1 showed a low removal of only 18.77%, compared to the other tested
concentrations. Furthermore, the data indicated that both the 1.0 mol
L-1 and 2.0 mol L-1 concentrations reached a plateau in
recent times, while the 0.125 mol L-1 concentration showed a linear
increase until the end, suggesting that, at longer times, the 0.125 mol
L-1 concentration can obtain higher COD removal values compared to
1.0 mol L-1 concentration.
During the treatment of the CNSL effluent, the H2 production in the
cathode system was determined simultaneously in volumes read at the same
predetermined times for the previously discussed COD analyses. The effects of
current density and electrolyte concentration on H2 production were
also evaluated. According to Figure 2a, it was observed that, when increasing
the current density, there is an increase in the production of H2.
The highest value of current density, 100 mA cm-2, reached a volume
of 1.9 L of H2, while the densities of 70 and 40 mA cm-2
reached hydrogen volumes of 1.28 and 0.73 L of H2, respectively. On
the other hand, as shown in Figure 2b, when evaluating the effect of NaOH
concentration with a fixed current density of 40 mA.cm-2, no large
variations in H2 production were observed, all conditions reached a
volume close to 0.75 L. Therefore, it can be suggested that the hydrogen
production is directly related to the applied current density, and the produced
volume presents a linear behavior in relation to time.
In addition, a brief analysis was made of the amount of energy that can be
recovered from the H2 produced in the treatment of the CNSL effluent,
as shown in Figure 3c, considering that 1 kg of H2 is equivalent to
39.9 kWh (Ivy, 2004). It was found that as the current density increases, the
energy production from H2 also increases. Therefore, the use of a
hybrid process for energy recovery in the treatment of effluents can be a viable
option to cover the energy costs of the process, making it more sustainable and
economically attractive. To maximize the energy yield of the process, future
research aimed at optimizing H2 production is of great relevance.
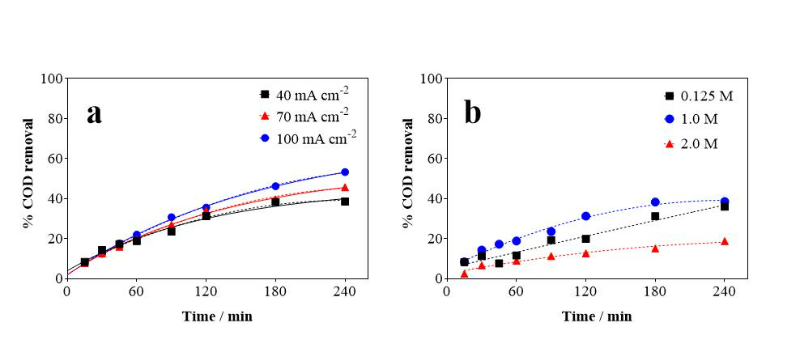
COD removal as a function of electrolysis time at \r\nroom temperature: (a) effect of current density and \r\n(b) effect of NaOH concentration.
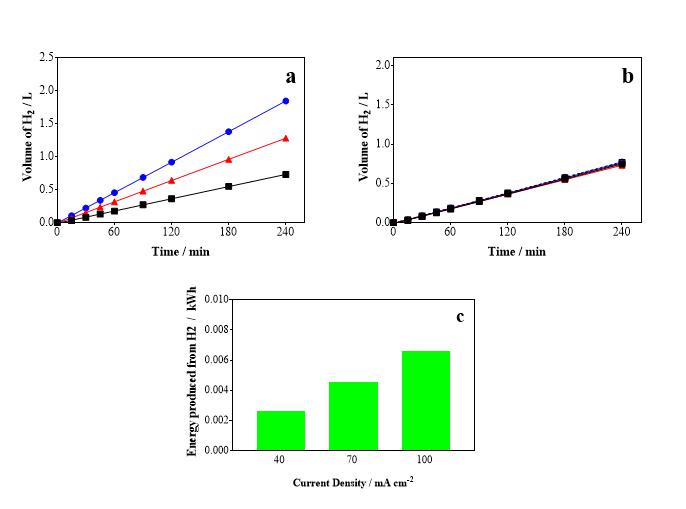
(a) Effect of current density; (b) Effect of NaOH \r\nconcentration; and (c) Amount of energy \r\ngenerated(kWh) from the production of H2.
Conclusões
From the obtained results, it can be concluded that the current density and electrolyte concentration are important factors that affect the efficiency of electrochemical oxidation in the treatment of CNSL effluents. It was observed that higher current densities and lower NaOH concentrations led to higher COD removal rates, although the 1 mol L-1 concentration of NaOH showed slightly greater degradation than that of 0.125 mol L-1 in the electrolysis time of 240 min. On the other hand, H2 production is directly related only to applied current density, with higher current densities resulting in greater volumes of H2 and power generation from the H2 produced. These findings suggest that a hybrid process for energy recovery in wastewater treatment can be a sustainable and economically attractive option, and further research aimed at optimizing H2 production is needed to maximize energy yield. Furthermore, in future research, the intermediates generated during the effluent oxidation process may contain an added value that can be recovered and contribute to the sustainability and cost recovery of the process, which is in line with the principles of circular economy.
Agradecimentos
For financial support from Conselho Nacional de Desenvolvimento Científico e Tecnológico (CNPq, Brazil) and Universidade Federal do Rio Grande do Norte and Universidade do Estado do Rio Grande do Norte.
Referências
CARDOZO J C, DA SILVA D R, MARTÍNEZ-HUITLE C A, QUIROZ M A, DOS SANTOS E V. Photovoltaic Electrochemically Driven Degradation of Calcon Dye with Simultaneous Green Hydrogen Production. Mater., v. 15, 2022.
HENRIQUE J M M, MONTEIRO M K S, CARDOZO J C, MARTÍNEZ-HUITLE C A, DA SILVA D R, DOS SANTOS E V. . Integrated-electrochemical approaches powered by photovoltaic energy for detecting and treating paracetamol in water. J. Electroanal. Chem. v. 876, 2020.
IVY J. Summary of Electrolytic Hydrogen Production: Milestone Completion Report. United States: Small, 2004.
MEDEIROS M C, DOS SANTOS E V, MARTÍNEZ-HUITLE C A, FAJARDO A S, CASTRO S S L. Obtaining high-added value products from the technical cashew-nut shell liquid using electrochemical oxidation with BDD anodes. Sep. Purif. Technol., v. 250, p. 117099, 2020.
MGAYA J, SHOMBE G B, MASIKANE S C, MLOWE S, MUBOFU E B, REVAPRASADU N. Cashew nut shell: A potential bio-resource for the production of bio-sourced chemicals, materials and fuels. Green Chem., v. 21, p. 1186–1201, 2019.
MILLER H A, BOUZEK K, HNAT J, LOOS S, BERNÄCKER C I, WEIßGÄRBER T, RÖNTZSCH L, MEIER-HAACK J. Green hydrogen from anion exchange membrane water electrolysis: a review of recent developments in critical materials and operating conditions. Sustain. Energy Fuels, v. 4, p. 2114–2133, 2020.
NOUSSAN M, RAIMONDI P P, SCITA R, HAFNER M. The Role of Green and Blue Hydrogen in the Energy Transition—A Technological and Geopolitical Perspective. Sustain, v. 13, p. 298, 2021.