ÁREA
Química Analítica
Autores
Meza López, F.L. (NATIONAL UNIVERSITY OF ENGINEERING,) ; Khan, S. (STATE UNIVERSITY OF SÃO PAULO (UNESP)) ; Lopez Cisneros, R. (NATIONAL UNIVERSITY OF ENGINEERING,) ; Picasso, G. (NATIONAL UNIVERSITY OF ENGINEERING,) ; Taboada Sotomayor, M.D.P. (STATE UNIVERSITY OF SÃO PAULO (UNESP))
RESUMO
This work reports published research of the development and application of a novel highly efficient ion imprinted polymer-based optical sensor for Pb ion determination. The sensor was synthesized by polymerization directly onto a quartz optical fiber. The polymerized fibers were characterized by FTIR and SEM. The results obtained under the optimized conditions employed were: linear response range of 10 to 100 μg L-1; linear regression coefficient of R2=0.9973 and detection limit of 85 ng L-1. The results obtained from reproducibility and repeatability studies showed RSD values below 4%. The studies carried out on the proposed sensor demonstrated the feasibility of its implementation and application in samples of environmental interest with recovery percentages of almost 100%.
Palavras Chaves
Lead ion; ion-imprinted polymer; optical sensor
Introdução
Lead, in its divalent Pb2+ form, is highly toxic at low concentrations; this is largely due to its ability to bioaccumulate in the organism of living beings that consume it, causing a wide range of disorders and diseases. There has been considerable interest among researchers in the field regarding the development of tools and techniques aimed at monitoring and studying the presence of lead in water bodies as a way of mitigating the risks posed by the pollutant to living beings and to the environment as a whole. Among the methods used for the detection of lead in water samples include atomic absorption spectroscopy (Uslu et al., 2018), inductively coupled plasma optical emission spectroscopy (ICP-OES) (Lingling et at., 2012), atomic fluorescence spectrometry (Qingxiang et al., 2011) and potentiometric methods (Isaiah et al., 2017); such as the validated analytical techniques used for the quantification of metal ions. One needs to point out, however, that the sophisticated nature of these techniques, coupled with some of their non-negligible limitations, especially in terms of costs, quantity and sample pretreatment, makes them impossible to be employed for on- site monitoring. The use of a wide range of sensors has been found to be promising for monitoring the presence of metal ions in water samples. Recent studies published in the literature have employed chemical and biological reagents as selective recognition phases for the construction of optical sensors, where the response of the sensors is determined by the change in some of their optical properties that can be measured or monitored; these properties include absorbance, reflectance, fluorescence, phosphorescence and evanescence properties, to name a few (Marcos et al., 2015). Heeyoung et al. (2011) reported to have developed a Pb2+ sensitive rhodamine fluorescent sensor using a self- assembled system, where they employed a wide range of reagents for this purpose. However, Jun et al. (2017) and Chun-Yan et al. (2013) showed that in general the use of fluorescence techniques for the detection of heavy metal ions faces some obstacles. Ali et al. (2020) and Tsao-Jen and Chung (2008) used optical fiber coated with nanoparticles for the detection of lead ions. As aforementioned, the determination of Pb ions has attracted considerable interest in the field of separation chemistry, and although materials supported on a surface or transducer can detect and adsorb Pb2+ ions with low detection limits, they require many and complicated synthesis processes, in addition to presenting low selectivity. One of the most promising approaches to the development of highly selective sensors is the application of ion recognition materials through ion imprint polymerization methods. Ion imprinted polymers (IIP) can selectively and efficiently yield good separation and recovery of important ions – especially metal ions, in different matrices (Mariana and Carla, 2017). Several studies published in the literature related to the construction of ion imprinted polymers (IIPs) have employed the same imprinting techniques that have been used for the construction of molecularly imprinted polymers (MIPs); these techniques are based on the reactions of functional monomers undergo polymerization in the presence of the template with a cross-linking reagent and a radical initiator (Catherine et al., 2013). The application of IIP materials in electrochemical sensors has been widely reported in the literature (Jaime et al., 2020). Other common strategies employed in the construction of sensors involve the incorporation of imprinted polymers in optical fibers, as reported by Bianca et al. (2020) and Marcos et al. (2015) who developed optical sensors using optical fibers as a transducer upon which a selective MIP for dyes. A thorough search into the literature showed that, to date, there have been few reports on the use of IIP as an optical device or sensor for the determination of metal ions. Interestingly, through a meticulous investigation of bibliographic reports on different reagents that act as chemosensors for Pb2+, we found studies that point to rhodizonate ion as a very sensitive and specific reagent for Pb2+ (Flor et al., 2021). The specific interaction between rhodizonate and lead can be used to obtain a new generation of IIPs, as well as previous study (Flor et al., 2021), which will be based on the intrinsic stability of the complex formed, thus enabling the application of the material in aqueous samples. Bearing this in mind, this work sought to develop a novel IIP-based optical sensor for the selective detection and quantification of Pb2+ in water samples. The proposed sensor is relatively less costly, easy to operate, and it is highly efficient for monitoring the contamination of water resources by metal ions. The novelty of this work lies in the development and application of an optical sensor produced from ion-imprinted polymer (IIP).
Material e métodos
Immobilization of the ion imprinted polymers (IIPs) on optical fiber: The immobilization of the IIPs on the optical fibers was carried out in a vial with 0.01 mmol of rhodizonate and 0.06 mmol of 2-acrylamido-2-methylpropane sulfonic acid (AMPSA) dissolved in water. Afterwards, 0.005 mmol of Pb(NO3)2 was added to the mixture; subsequently, 0.6 mmol of N,N-methylene bisacrylamide (MBA) and 0.01 mmol of potassium persulfate (KPS). Finally, the silanized optical fiber (previously treated) was placed in the vial. The polymerization was carried out at 70 °C for 24 h in an inert environment of N2. For the elimination of the metal ion, a solution of 1.0 mol L-1 HNO3 was used. The NIP was synthesized in the same manner as the IIP but in the absence of Pb(NO3)2. Characterization: The materials developed in this study were characterized by Fourier transform infrared spectroscopy (FTIR) in the range of 4000-400 cm-1 using a Bruker Vertex 70 spectrophotometer. The morphology of the optical fibers was characterized by field emission gun scanning electron microscopy (FEG-SEM) using a JEOL microscope model 7500F. The tip sensors were attached to a bundle of Agilent® optical fibers Cary 60 model) - acquired from Agilent Technologies®-coupled to a UV/Vis spectrophotometer. The spectrophotometer was controlled using the ScanCaryWinUV® software. To contrast the results obtained, the analytical determination of Pb ion was conducted at λ=217.0 nm in air/acetylene flame using Analytik Jena ContrAA 300 high-resolution continuum source atomic absorption spectrometer (Jena, Germany). Optimization of the optical sensor response: The fibers immobilized with the polymeric materials and coupled to a bifurcated bundle of optical fibers were incorporated into a UV/Vis spectrophotometer, obtaining the optical sensor. For the conduct of the reflectance measurements, the laboratory protocol was strictly adhered to. First, the optode was inserted into a solution containing the analyte under optimized time and pH. After the interaction of the Pb ion with IIP-optode or NIP-optode, the optical sensor was removed from the solution and the device was washed to remove any excess solution. To perform the reflectance measurements, the optode was immersed in a vial containing water and the measurements were carried out; here, maximum absorbance at 535 nm was defined as characteristic of the interaction between rhodizonate and Pb ion. The optical sensors were evaluated under the previously established optimal conditions of pH of the solution and interaction time aiming at estimating the limit of detection (LOD), limit of quantification (LOQ), repeatability, reproducibility and the relative standard deviation (RSD). As part of the study of the practical efficiency of the optical sensor, an analysis was conducted aiming at assessing the applicability of the sensor in real samples (Batalha, Guaçu and Pepira Rivers).
Resultado e discussão
Characterization:
The FTIR analysis shows the most characteristic stretching vibrations of the IIP-
optode and NIP-optode. The absence of the vibration band related to the vinyl
group C=C at 1616 cm-1 of the MBA spectrum in the polymeric materials and the
inexistence of the C-H bond with sp3 hybridization at 2930 cm-1 confirm the
occurrence of complete polymerization process (Catherine et al., 2013; Chun-Yan
et al., 2013). The SEM micrographs of the fibers immobilized with IIP one will
observe the presence of more distributed and homogeneous particles adhered to the
optical fiber.
Optimization of the sensor response:
A study was conducted in order to investigate the effect of the pH and the
interaction time between the Pb2+ and the sensor. Experiments revealed that the
best time for an effective detection of Pb2+was found to be 10 minutes, since at
higher periods of time, the response sensor was found to be constant. Also the
analytical curve obtained at pH 6 for the IIP-optode presented the greatest
variation in reflectance, and consequently a better detection response of Pb ion.
The response profile of the optical sensor:
An analysis was conducted aiming at evaluating the response profile of the sensor
under the previously established optimal conditions of pH and interaction time.
For this analysis, analytical curves were constructed at different concentrations
of Pb2+. The values related to LOD and LOQ, as well as linear range and
sensitivity were calculated. Figure shows the defined variation of reflectance
measured in the IIP-optode at different analyte concentrations. As can be
observed, unlike the NIP-optode, the imprinted polymer immobilized on the optical
fiber was found to have efficiently adsorbed the analyte. The analytical curve of
the IIP-optode presented adequate linearity between 10 – 100 μg L-1, with a
regression coefficient of R2 = 0.9973. Moreover, the use of the IIP-optode led to
a more sensitive detection compared to the NIP-optode. The limit of detection
(LOD) obtained for the IIP-optode was 85 ng L-1; this shows that the imprinted
polymer has good sensitivity. The detection limit obtained for the proposed
sensor was compared with that of other sensors reported in the literature.
It is worth noting that, the proposed IIP-optode sensor presented a limit of
detection lower than that of almost all the sensors reported in the literature to
date (Heeyoung et al., 2011; Thiphol et al., 2016; Jun et al., 2017; Suraya et
al., 2018; Ali et al., 2020). The study of repeatability is aimed at evaluating
the agreement between the results obtained successively via the application of
the same methods under the same measurement conditions (Bianca et al., 2020). To
conduct this analysis, seven consecutive measurements were performed in order to
construct analytical curves for the IIP-optode and the NIP-optode using the same
measurement conditions at 535 nm. The equations related to the analytical curves
of the IIP-optode and NIP-optode with regression coefficient of 0.9973 and
0.5244, respectively, exhibited a relative standard deviation (RSD) below 1%;
this indicates good and promising results.
The reproducibility of the proposed sensor was evaluated using three different
IIP-optodes and three different NIP-optodes prepared under the same previously
described conditions. The results obtained show that the three fibers recorded a
relative standard deviation (RSD) of less than 3%; this points to a high degree
of agreement between the measurements of the three fibers, based on the
analytical curves obtained and the linear adjustments carried out with values of
linearity coefficients (R2) of 0.9946 and 0.7956 for the IIP-optode and the NIP-
optode, respectively.
Selectivity study: To study the selectivity of the proposed sensor in terms of Pb
detection, the sensor response was evaluated in the presence of interfering ions
with similar properties (Ni2+, Cu2+, Cd2+, Zn2+, Co2+ and Fe2+). Different
amounts of the interfering ions were added to Pb solutions and an analytical
curve in the same response range was generated based on the same analytical
methodology. Each metal ion was analyzed at its specific wavelength by
reflectance, which was determined experimentally. The response of the IIP-optode
in the presence of interfering ions shows that the reflectance band for Pb at 535
nm was not affected by the presence of the interfering ions; furthermore, any
reflectance peak in the visible region which corresponded to the interfering ions
was only observable at wavelength values below 400 nm. The analytical curve
obtained showed adequate linearity (R2 = 0.9925). The results obtained point to
the selectivity of the IIP-optode sensor for Pb2+ detection in the presence of
other metal ions due to the absence of reflectance bands in the visible region.
Apart from being related to the reflectance spectra obtained by the IIP-optode in
contact with the interfering metal ions separately, the bands observed between
200-400 nm corresponded to the chromophore groups present in the ligand and
monomers that make up the imprinted polymer the groups include the saturated
amino and sulfonic groups of the functional monomer (λmax=220), and the enol and
carbonyl groups of the ligand (λmax 310-325) (Anthony, 2013). These functional
groups generate an electronic transition (n → π*), which leads to the production
of an absorption in the near range of the UV spectrum, thus contributing toward
the generation of the reflectance peaks observed in these regions. To numerically
validate the selectivity of the proposed sensor, the selectivity factor (β) was
evaluated using the equations based on the molecular impression factor (α) which
relate the reflectance variation between the IIP-optode and the NIP-optode
(Bianca et al., 2020). The values obtained for β were significantly higher, and
this points to a strong selectivity of the IIP-optode toward Pb ion. The greater
rebinding affinity of the IIP-optode can be associated with the imprinted
cavities and specific binding sites formed in the imprinted polymer. Positioned
in a predetermined orientation, these specific sites form well-designed
coordination geometry for the rhodizonate ligand; in other words, the IIP-AMPSA
preferred chelation with the Pb2+ in the presence of other metals due to the
successful imprinting process (Flor et al., 2021).
The applicability and detection capacity
of the proposed sensor in real samples were evaluated using water samples
collected from three rivers - Batalha, Guaçu, and Pepira rivers, located in the
State of São Paulo – Brazil. The recovery percentage (percentage measure of the
interaction between the analyte and optode) was calculated in order to evaluate
the performance of the optodes in real sample matrices. The results obtained were
contrasted by flame atomic absorption spectroscopy (FAAS) analysis, according to
the methodology described previously. Based on the results, the samples from the
Batalha, Guaçu and Pepira rivers presented traces of Pb of approximately 110, 30
and 40 μg L-1, respectively. Through the application of the IIP-optode, the
recovery percentage values obtained for all the river water samples analyzed were
close to 100 %. The results obtained from the analyses conducted on the sensor
developed here based on Pb ion-selective IIP optode showed the viability of the
development and application of the device in samples of environmental interest.
The polymeric material used for obtaining the optical sensor exhibited a recovery
efficiency of almost 100 %; this indicates a strong anti-interference ability in
real water samples and points to the high efficiency of the proposed sensor for
the detection of Pb2+.
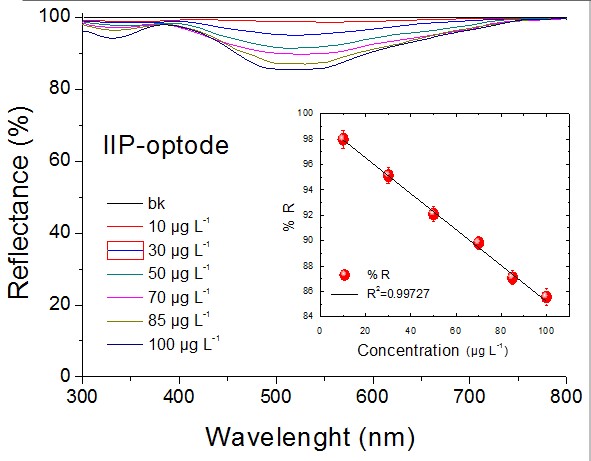
Reflectance spectra in the UV-Vis region at different \r\nPb2+ concentrations obtained for the IIP-optode under \r\noptimized conditions: pH 6 and 10 min.
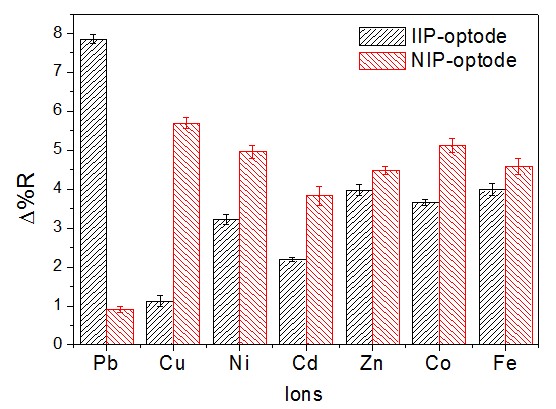
Variation of the percentage of reflectance for \r\ndifferent metal ions obtained under the application of \r\nthe IIP-optode and the NIP-optode.
Conclusões
The present work reported the successful development of a novel optical sensor based on immobilized fibers using polymeric materials and its application for Pb detection in water samples. The response profile of the optical sensor was found to be highly efficient and the device exhibited excellent selectivity for Pb detection in the presence of interfering ions – the selectivity of the device was attributed to the shape of the cavities formed. Analytical curves were constructed under optimized conditions where a limit of detection of 85 ng L-1 was obtained. The figures of merit presented low RSD; this pointed to the good sensitivity and selectivity of the sensor as a suitable alternative device for the detection and quantification of Pb ion. The results obtained from the analyses conducted here helped confirm the viability of the implementation of the optical sensor and its application in samples of environmental interest.
Agradecimentos
The authors are grateful to CONCYTEC (grant #PE501080603-2022-PROCIENCIA), FONDECYT- BC (grant #227-2018- FONDECYT) and FAPESP (grants #2014/50945-4 and #2019/00677-7) for the financial assistance.
Referências
ALI, A. A., SARMAD, F. A., MOHD, H. Y., HONG, N. L., MOHD, A. M., 2020. Surface Plasmon Resonance Sensor based on D-Shaped optical fiber using fiberbench rotating wave plate for sensing Pb ions. Optik, 202, 163724.
ANTHONY, T., 2013. Biosensors: sense and sensibility. Chem. Soc. Rev. 42, 3184.
BIANCA, M., SABIR, K., ADEMAR, W., ROSA, F. D., MARIA D. P. T. S., 2020. Next generation of optodes coupling plastic antibody with optical fibers for selective quantification of Acid Green 16. Sensors and Actuators: B. Chemical. 305, 127553.
CATHERINE, B., WALID, M., ANDRE, M., 2013. Recent advances on ion imprinted polymers. Reactive and Functional Polymers. 73 (6), 859-875.
CHUN-YAN, L., YU, Z., YONG-FEI, F., XUE-FEI, K., CHUN-XIANG, Z., CHAO, W., 2013. Colorimetric and fluorescent chemosensor for citrate based on a rhodamine and Pb2+ complex in aqueous solution. Analytica Chimica Acta. 774, 79-84.
FLOR, D. L. M. L., SABIR, K., MATHEUS, A. S., JOSÉ, A. G. N., GINO, P., MARÍA, D. P. T. S., 2021. Systematic study on the synthesis of novel ion-imprinted polymers based on rhodizonate for the highly selective removal of Pb (II). Reactive Functional Polymers. 159, 104805.
HEEYOUNG, J., MIN, H. L., JUNGAHN, K., JONG, S. K., JOOHOON, K., 2011. Rhodamine-based chemosensing monolayers on glass as a facile fluorescent “turn-on” sensing film for selective detection of Pb2+. Talanta. 83, 1359–1363.
ISAIAH, O., SALIHU, L., ABUBAKAR, I., EZEKIEL, F., JUSTINAH, A., 2017. Removal of lead ions from water and wastewaters electrochemically. Water Purification. 643–691.
JAIME, P., RODRIGO, S., DIEGO, T., FREDDY, N., FRANCESCA, F., MARÍA J. A., 2020. Inexpensive and green electrochemical sensor for the determination of Cd (II) and Pb(II) by square wave anodic stripping voltammetry in bivalve Mollusks. Food Chemistry. 321, 126682.
JUN, W., KUN, K., CHAO, L., YUYUAN, L., SHUYAN, N., 2017. A novel fluorescent chemosensor based on a rhodamine 6G derivative for the detection of Pb2+ ion. Sensors and Actuators B: Chemical. 246, 696-702.
LINGLING, Z., SHUXIAN, Z., KEMING, F., ZHAOSHENG, Q., JIANRONG, C., 2012. Determination of cadmium (II), cobalt (II), nickel (II), lead (II), zinc (II), and copper (II) in water samples using dual-cloud point extraction and inductively coupled plasma emission spectrometry. J. Hazard. Mater. 239-240, 206-212.
MARCOS, V. F., XUAN-ANH, T., MARIA, V. B. Z., MARIA, D. P. T. S., KARSTEN, H., BERNADETTE, T. S. B., 2015. A molecularly imprinted polymer-based evanescent wave fiber optic sensor for the detection of basic red 9 dye. Sensors Actuators B Chem. 218, 222–228.
MARIANA, R. G., CARLA, G. B., 2017. Molecularly imprinted polymers for bioanalytical sample preparation. Journal of Chromatography B. 1043, 107-121.
QINGXIANG, Z., NA, Z., GUOHONG, X., 2011. Determination of lead in environmental waters with dispersive liquid-liquid microextraction prior to atomic fluorescence spectrometry. J. Hazard. Mater. 189, 48-53.
SURAYA, A., NUR, H. A., NADHRATUN, N. M., MOHD, S. D. Z., AHMAD, A. A. B., 2018. Sensitivity enhancement of localized SPR sensor towards Pb(II) ion detection using natural bio-polymer based carrageenan. Optik. 168, 784-793.
THIPHOL, S., JUWADEE, S., ATITAYA, S., 2016. Paper-based analytical device for sampling, on-site preconcentration and detection of ppb lead in water. Talanta. 154, 504-510.
TSAO-JEN, L., CHUNG, M. F., 2008. Using monoclonal antibody to determine lead ions with a localized surface plasmon resonance fiber-optic biosensor. Sensors. 8, 582–593. DOI: 10.3390/s8010582.
USLU, H., BÜYÜKPINAR, Ç., UNUTKAN, T., SERBEST, H., SAN, N., TURAK, F., BAKIRDERE, S., 2018. A novel analytical method for sensitive determination of lead: Hydrogen assisted T-shape slotted quartz tube-atom trap-flame atomic absorption spectrometry. Microchemical Journal. 137, 155-159.