Autores
Salazar, J. (UNIVERSIDADE FEDERAL FLUMINENSE) ; Navas, G. (UNIVERSIDADE FEDERAL FLUMINENSE) ; Moreira, M. (UNIVERSIDADE FEDERAL FLUMINENSE) ; Nóbrega, G. (UNIVERSIDADE FEDERAL FLUMINENSE) ; Albuquerque, A.L. (UNIVERSIDADE FEDERAL FLUMINENSE) ; Bahr, A. (HEIDELBERG UNIVERSITY) ; Díaz, R. (UNIVERSIDADE FEDERAL FLUMINENSE)
Resumo
Was study the tailing influence from SAMARCO’s Company on the marine sediment
dynamics from Doce river (DR) discharge zone, evaluating the geochemistry of
sulfur, iron and manganese, comparing with the dynamics of the marine sediments
not affected by tailings like Jequitinhonha river (JR) discharge zone. Sulfur, Fe
and Mn were studied by quantifying the Acid Volatile Sulfide (AVS) and Chromium
Reducible Sulfur (CRS), as well as Degree of Pyritization of iron (DOP) and Fe and
Mn sedimentary fractionation. Was determined that in DR, there are favorable redox
conditions for AVS and pyrite formation and Fe and Mn concentrations are higher
than those reported for JR, indicating a supply of these metals by the tailings.
Furthermore, the Fe supply favored the primary productivity increase in DR.
Palavras chaves
Pyritization; Tailling; Metal
Introdução
The main sediment source for the marine environment is river transport, which is
responsible for almost 90% of the sedimentary contribution to coastal
environments. At Coastal zones represent 18% of the total land surface, and
approximately 44% of the world population depends directly or indirectly on
coastal zones for its development (RAJARAM AND GANESHKUMAR, 2015; BASTOS et al.,
2017). However, industrial and urban activities contributed to the contamination
of coastal marine environments, having an impact in coastal ecosystems. In fact,
sediments influenced by industrial activities have a high content of metals and
can be responsible for increases in the sedimentation rate and organic matter
contribution (HUERTA-DÍAZ and MORSE, 1990; TESSIER et al., 1996; BUCCOLIERI et
al., 2006; RAJARAM AND GANESHKUMAR, 2015).
In marine sediments, metals can have their bioavailability controlled by the
mineralogical composition, the conditions redox of the medium and the content of
organic matter (BUCCOLIERI et al., 2006; KAUSHIK et al., 2009; KALNEJAIS et al.,
2015). Thus, when the redox condition of the sedimentation changes and there is
a decrease in dissolved oxygen concentration (sub-oxic or anoxic condition - due
to increased primary production or the rate of sedimentation) the trace metals
are released from the oxyhydroxides (QUEIROZ et al., 2021b) and can interact
with reactive organic matter, clays, with dissolved sulfide in the middle or
enter the water column, affecting the water quality and resulting in risk to
biota (BUCCOLIERI et al., 2006; YANG et al., 2009).
On the Brazilian East coast, the Doce River is one of the primary sediment
source to the continental shelf. However, on November 5th 2015, the worst
environmental accidents in the history of Brazil occurred when the “Fundão”
tailings dam collapsed (Minas Gerais state, SE-Brazil). As a result, the
tailings reached the rivers “Gualaxo do Norte” (over 55 km), “Carmo” (over 22
km), and “Doce” (over 600 km), until reaching the Atlantic Ocean on November
22nd on the coast of Espírito Santo state (SE-Brazil). The collapse contributed
to 60x106 m3 of tailings to the coastal zone, composed of heterogeneous material
with high amounts of crystalline Fe oxyhydroxides (MARTA-ALMEIDA et al., 2016,
QUEIROZ et al., 2021a).
Consequently, the mining tailings’ arrival on the continental shelf may have
increased iron, manganese, trace metals, and organic matter, modifying the
dynamics of marine sediments. In this sense, Fe and Mn, which are important
constituents of the mine tailings, have their bioavailability and behavior
controlled by the redox conditions and organic matter content (BUCCOLIERI et
al., 2006, KAUSHIK et al., 2009, BERNER, 1984, KALNEJAIS et al., 2015). Thus, as
oxidation potential (Eh) decreases, trace metals are released from the
oxyhydroxides (QUEIROZ et al., 2021b), which may react with organic matter, clay
minerals, and dissolved sulfide or enter into the water column, increasing the
risk to the biota (BUCCOLIERI et al., 2006, YANG et al., 2009).
Thus, in works carried out in the Doce River drainage basin in order to
determine the presence of trace metals and metalloids in samples of sediments
from the river bottom, estuary, and river mouth, as well as in the estuarine
soils, were observed an increase in metals contents such as Cr, Co, Cu, Fe, Hg,
Pb and Zn after the arrival of tailings. In addition, increased contents in Mn a
P in the estuary soils were also reported, as well as Mn increased concentration
in fishes (TYRRELL, 1999, SANTOLIN et al., 2015, HATJE et al., 2017, QUEIROZ et
al., 2018a, D′AZEREDO ORLANDO et al., 2020, DOS REIS et al., 2020, QUEIROZ et
al., 2021a, QUEIROZ et al., 2021b). On the other hand, changes in the
sedimentation environment redox conditions might favor the trace metals release
, considered toxic, as well as phosphorus, which is an important nutrient and
can directly affect the environment primary productivity (TYRRELL, 1999, QUEIROZ
et al., 2021a). However, few works evaluated the impacts of tailings discharge
on the coastal shelf. In this context, this work evaluated the sedimentary
geochemistry of inorganic sulfur species: Acid Volatile Sulfide - AVS
(Volatilizable Sulfides by Acidification) and Chromium Reducible Sulfur - CRS
(Chromium Reducible Sulfur (CRS), as well as the fractionation of Mn and Fe,
assessing the degree of pyritization of Fe (DOP), to evaluate the mining
tailings effect on the redox condition of the sedimentation environment,
assessing the bioavailability of iron and manganese in the sediments from Doce
river discharge zone, compared with the discharge zone of a river not impacted
by large amounts of tailings but affected by mining activities, like the
Jequitinhonha river, which is an important natural resource affected by
agrilculture and mining activities.
Material e métodos
The marine sediment cores (M125-39-2 and M125-67-3) was collected on the
continental shelf, in the Doce and Jequetinhonha river discharge zone
respectively, during the research vessel R/V Meteor trip number M125, using a
“MUC” sediment multi-collector, as described in the report by BAHR et al.
(2016). The depth and length of the cores were 24 m / 29 cm and 28.2 m / 34 cm
respectively. Each core was sliced every 1 cm: part of the wet sediment was
stored for further analysis, and the other part was preserved in zinc acetate to
avoid sulfides oxidation.
Acid volatile sulfides (AVS) and Chromium-reducible sulfide (CRS) were extracted
using 1g of wet samples (preserved in zinc acetate), in an inert atmosphere, by
a two-step distillation: (I) AVS extraction, carried out at room temperature
with HCl (6M) during one hour (1h), where the released H2S was precipitated as
zinc sulfide (ZnS) in a flask tube containing zinc acetate (Zn(CH3COO)2 - 5%).
The second step (II) consisted of CRS extraction, at a temperature of 80 °C,
adding CrCl2 (2M) and leaving during one hour (1h), and the released H2S was
also precipitated as ZnS in a second 5% zinc acetate trap (FOSSING and
JØRGENSEN, 1989, PRAHARAJ and FORTIN, 2004). The distillations for each sample
were done in duplicates, with a variation coefficient lower than 15%.
The sulfide concentration was measured using a Thermo Scientific model Genesys
10 UV spectrophotometer (with a wavelength of 670 nm), previously adding N,N-
dimethyl-p-phenylenediamine (DMFD or Diamine) to the sample as a colorimetric
reagent (CLINE, 1969, KOLLURU et al., 2013).
The sequential extraction method proposed by HUERTA-DIAZ and MORSE (1990) was
used, obtaining two operationally defined fractions: reactive and pyritic.
Initially, the samples were lyophilized, macerated, and sieved (0.15 mm mesh).
Briefly, the reactive fraction was extracted using HCl 1M (20ml and agitated for
16 h, and the extract was collected after centrifugation. To the residue from
the HCl extraction, HF 10 M (30ml) was added agitated for 1h, and the extract
was collected after centrifugation. Subsequently, an additional HF 10 M (30ml)
was added and agitated for 16 h, than H3BO3 (5g) was added and agitated for 8 h
and, again, the extract was collected after centrifugation and the extract was
combined with the first HF extraction. The solid residue from the previous
extraction was treated with concentrated H2SO4 (10ml) and left under agitation
for 2 h to remove the organic matter. Finally, the extraction of the pyrite
fraction was performed by adding concentrated HNO3 (10ml) to the sediment
residue and shaking for 2 h. Between each step of the extraction procedure,
samples were washed with Milli-Q water.
All extracted fractions were analyzed using the Optical Emission Spectrometry
with an Inductively Coupled Plasma source (ICP - OES) at the School of
Agriculture “Luiz de Queiroz” of the University of São Paulo.
Resultado e discussão
TOC, AVS, CRS, and ST
TOC contents along the entire depth ranged between 1.04% and 1.96% for Doce
river (DR) and 1.64 – 2.02 % for Jequitinhonha river (JR), with a mean of 1.39 ±
0.26% and 1,86 ± 0,09 % respectively. The TOC content for DR, showed a slight
increase tendency with depth, specifically from 19 cm depth, where the value
ranges from 1.13% to 1.61%, while JR did not show variations with depth.
The TOC behavior throughout the DR core can be associated with the increase in
the input of material from the dam to the coastal environment, favoring the
increment in bacterial activity, as a consequence of the high inputs of Fe,
which is a micronutrient that favors the cyanobacterial community increase
(O’NEIL et al., 2012, VAALGAMAA et al., 2013). Additionally, according with
QUEIROZ et al. (2021a), the tailing contributed with a large amount of P to Doce
river coastal area, since the Fe and Mn oxyhydroxides also adsorbed high amounts
of P, which also favored the primary productivity and affecting the TOC
behavior.
Furthermore, the increment in primary production and subsequent degradation of
organic matter, preferably in the DR core upper layers, may have influenced in
the lower TOC compared to deeper layers.
Likewise, ST concentrations ranged from 0.05% to 0.46% for DR and 0.13 to 0,27%
for JR. With a tendency to decrease with depth for both cores. On the other
hand, the DR core presented an AVS average of 0.02 ± 0.024%, with lowest and
highest values of 4.2x10-6 % and 0.073 %, respectively. Thus, presenting two
critical accumulation zones at 18 and 25 cm deep. While JR core presented AVS
average values of 0.01 ± 0.005%.
In the case of CRS content, the mean was 0.06 ± 0.07% for DR core with minimum
and maximum concentrations of 0.01 and 0.25% respectively, where a tendency to
increase concentration with depth was found, from depth 21 cm. On the other
hand, JR core presented average values for CRS of 0.03 ± 0.02%, with minimum of
0.002% and maximum of 0.016%.
The tendency of TS and pyrite (CRS) to increase with depth is expected in DR
core, since the microbial degradation of organic matter through sulfate-
reduction reactions produce H2S and subsequently reacts with reactive iron, in
environments with low oxygen concentrations (BERNER, 1984, CANFIELD et al.,
1992, DÍAZ et al., 2012, JØRGENSEN et al., 2019), therefore, favorable redox
conditions for sulfide formation can be inferred; with iron hydroxides from the
tailing acting like rective Fe source to form iron sulfides. Furthermore, the
lower concentration of CRS compared to TS suggests that a sulfur fraction was
incorporated into organic matter (LÜCKGE et al., 2002).
Whereas, the first zone of accumulation of AVS at 18 cm for DR core may be
associated with the reactive iron arrival and its subsequent sulfurization,
indicating in turn the taillings influence on the sediments (QUEIROZ et al.,
2018b).
The second section of an accumulation of AVS at greater depth (about 26cm
depth), in agreement with the results obtained by WIJSMAN et al. (2001), can be
related to AVS that have not yet been transformed into pyrite (WIJSMAN et al.,
2001). Furthermore, AVS can be preserved in recent sediments as a consequence of
rapid sedimentation (BOESEN and POSTMA, 1988, GAGNON et al., 1995, MIDDELBURG,
1991), as reported in Kau bay, Indonesia (MIDDELBURG, 1991) and Saguenay Fjord,
Canada (GAGNON et al., 1995) and probably the Doce River discharge zone, as a
consequence of the tailings arrival, which increased suspended particulate
material (SPM) from 100 mg L-1 to 9000 mg L-1, according to CPRM (2015) and
QUARESMA et al. (2020).
Finally, the JR core, even with a TS higher concentration, the AVS absence
allows to infer that there is no significant contribution of reactive iron to
the environment.
Fe and Mn Behaviour
The highest Fe contents for DR core were found in the reactive fraction, with an
average concentration of 5,242.55 ± 1,213.30 mgkg-1 and higher than Fe conten in
pyrite fraction (384.24 ± 464.64 mg kg-1), with an increasing with depth for
both fractions. In JR core, the content average Fe was lowest for reactive
fraction (4,483.16 ± 239.58 mg kg-1) and higher for pyrite fraction (1,066.08 ±
709.76 mg kg-1) than the DR core, increasing with depth for pyrite fraction.
For Mn, the highest contents were found in the reactive fraction for DR and JR
cores (average of 294.83 ± 162.53 mg kg-1 and 166.46 ± 10.75 mg kg-1
respectively), with a tendency to increase with depth in DR core. The pyrite
fraction presented the lowest mean values (0.08 ± 0.09 mg kg-1), without
variations with depth.
This results suggest that significant amounts of iron oxyhydroxides associated
with tailing were delivered after dam rupture, which reflected in the high Fe
contents found in DR core. Besides, the Fe pyritic had a tendency to increase
its concentration with depth, evidencing an existing pyrite formation process
(HUERTA-DIAZ and MORSE, 1992, DÍAZ et al., 2012, MOREIRA et al., 2018). However,
the lower concentrations of Fe pyritic compared to Fe reactive, could be
associated with AVS that has not yet been transformed into pyrite (WIJSMAN et
al., 2001).
Furthermore, the reactive iron vs AVS showed two different groups of sediments
for Doce river: 0 - 20 cm and 20 - 34 cm, evidenced by the different trends. In
the deeper layer, the Fe decrease with the AVS increase is an expected behavior,
since it evidences the monosulfides formation from the reaction of iron with
sulfide. While, in the more superficial layer, the tendency of AVS increase with
the increase of reactive iron, indicates that even with the formation of
monosulfides there is no depletion of Fe, this as a consequence of the constant
supply of reactive iron to the environment during the taillings.
In the case of Mn, the highest concentrations found in the reactive fraction are
consistent with data obtained by OTERO et al. (2003), YE et al. (2010) and
MOREIRA et al. (2018). Which are a product of the fast reaction kinetics, which
favored its incorporation into carbonates and oxyhydroxides present in the
reactive fraction; subsequently, this fast reaction kinetics did not allow a
greater incorporation into pyrite (MORSE and LUTHER, 1999).
Both Fe and Mn are mainly linked to a reactive fraction, which are potentially
bioavailable. However, if the environmental conditions change to more reducing
environments, they can be released from the oxyhydroxides and increasing its
bioavailability in the environment (QUEIROZ et al., 2021b, MORSE, 1994, ÁLVAREZ-
IGLESIAS and RUBIO, 2008). Besides, Mn in high concentrations presents a risk as
a toxic element for different aquatic species. In fact, according with QUEIROZ
et al. (2021b), was found a possible chronic contamination scenario within the
Doce river estuary, suggesting a high risk to estuarine biota and human.
Finally, the DOP presented values ranged range of 0.41% - 17.78% and 0.33 –
29.75 for DR and JR cores respectively,. Furthermore, the trend along the core
was to increase the DOP value with depth for both cores, especially from the 20
cm layer of the DR core. The degree of pyritization (DOP) average (5.5%) of DR
core indicates an environment with oxic conditions according to RAISWELL et al.
(1988); which makes sense with the smallest concentrations of Fe pyritic respect
to Fe reactive.
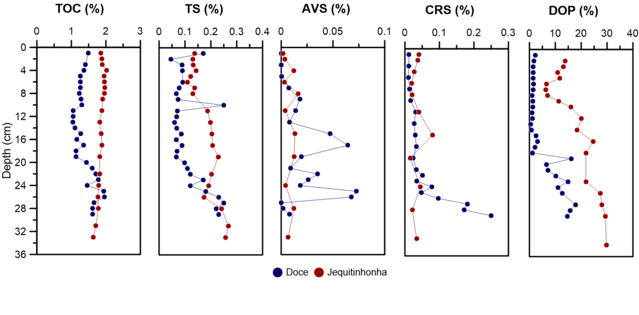
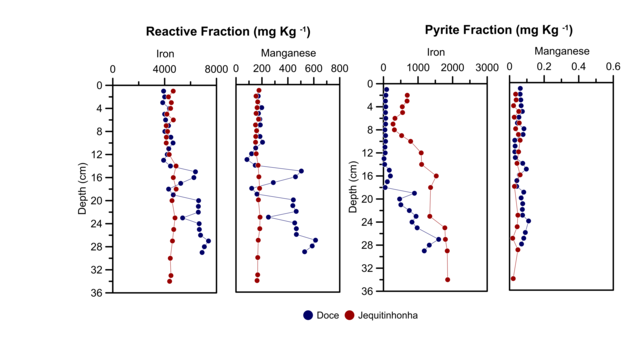
Conclusões
The arrival of mining tailings to the Rio Doce discharge zone, as a consequence of
the Fundão dams rupture, brought high concentrations of Fe and Mn, which favored
the increase in the primary productivity of the environment and, therefore, the
behavior of TOC, mainly in the most superficial layers.Since this Fe and Mn is
mainly associated with the more reactive fractions found in the sediments and
encounters above those found in marine sediments from the east bank of Brazil,
such as the discharge zone of the Jequitinhonha River, evidencing the contribution
of these metals by the arrival of the mining tailings.
Furthermore, changes in the redox conditions of the medium favored the reduction
of marine sulfate to sulfide, which consequently formed iron sulfides (AVS and
CRS), where iron monosulfides (AVS) are evidence of the arrival of mining tailings
and its effect. on the dynamics of the sediments present at the mouth of the Rio
Doce.
On the other hand, the high concentrations of Mn present in the reactive fraction
of the sediments present a potential risk for the biota, mainly fish, which could
incorporate this metal in their tissues as reported in the estuary of the Rio
Doce.
Agradecimentos
Thanks to the support by CAPES and FAPERJ, the Geosciences Program (Geochemistry)
from Universidade Federal Fluminense (UFF) and Anthropocene Geochemistry and
Oceanography and Paleoceanography laboratories from UFF.
Referências
ÁLVAREZ-IGLESIAS, P. & RUBIO, B. 2008. The degree of trace metal pyritization in subtidal sediments of a mariculture area: Application to the assessment of toxic risk. Marine Pollution Bulletin, 56, 973-983.
BAHR, A., ALBUQUERQUE, A., ARDENGHI, N., BATENBURG, S., BAYER, M., A CATUNDA, M., CONFORTI, A., DIAS, B., DÍAZ, R., EGGER, L., EVERS, F., FISCHER, T., HATSUKANO, K., HENNRICH, B., HOFFMANN, J., JIVCOV, S., KUSCH, S., MUNZ, P., NIEDERMEYER, E. & WACHHOLZ, A. 2016. South American Hydrological Balance and Paleoceanography during the Late Pleistocene and Holocene (SAMBA) - Cruise No. M125 - March 21 - April 15, 2016 - Rio de Janeiro (Brazil) - Fortaleza (Brazil).
BERNER, R. A. 1984. Sedimentary pyrite formation: An update. Geochimica et Cosmochimica Acta, 48, 605-615.
BOESEN, C. & POSTMA, D. 1988. Pyrite formation in anoxic environments of the Baltic. American Journal of Science, 288, 575.
BUCCOLIERI, A., BUCCOLIERI, G., CARDELLICCHIO, N., DELL'ATTI, A., DI LEO, A. & MACI, A. 2006. Heavy metals in marine sediments of Taranto Gulf (Ionian Sea, Southern Italy). Marine Chemistry, 99, 227-235.
CANFIELD, D., RAISWELL, R. & BOTTRELL, S. H. 1992. The reactivity of sedimentary iron minerals toward sulfide. American Journal of Science, 292.
CLINE, J. D. 1969. SPECTROPHOTOMETRIC DETERMINATION OF HYDROGEN SULFIDE IN NATURAL WATERS1. Limnology and Oceanography, 14, 454-458.
D′AZEREDO ORLANDO, M. T., GALVÃO, E. S., SANT′ANA CAVICHINI, A., GABRIG TURBAY RANGEL, C. V., PINHEIRO ORLANDO, C. G., GRILO, C. F., SOARES, J., SANTOS OLIVEIRA, K. S., SÁ, F., JUNIOR, A. C., BASTOS, A. C. & DA SILVA QUARESMA, V. 2020. Tracing iron ore tailings in the marine environment: An investigation of the Fundão dam failure. Chemosphere, 257, 127184.
DÍAZ, R., MOREIRA, M., MENDOZA, U., MACHADO, W., BÖTTCHER, M., SANTOS, H., BELEM, A., CAPILLA, R., ESCHER, P. & ALBUQUERQUE, A. 2012. Early diagenesis of sulfur in a tropical upwelling system, Cabo Frio, southeastern Brazil. Geology, 40, 879-882.
DOS REIS, D. A., NASCIMENTO, L. P., DE ABREU, A. T., NALINI JÚNIOR, H. A., ROESER, H. M. P. & DA FONSECA SANTIAGO, A. 2020. Geochemical evaluation of bottom sediments affected by historic mining and the rupture of the Fundão dam, Brazil. Environmental Science and Pollution Research, 27, 4365-4375.
FOSSING, H. & JØRGENSEN, B. B. 1989. Measurement of bacterial sulfate reduction in sediments: Evaluation of a single-step chromium reduction method. Biogeochemistry, 8, 205-222.
GAGNON, C., MUCCI, A. & PELLETIER, É. 1995. Anomalous accumulation of acid-volatile sulphides (AVS) in a coastal marine sediment, Saguenay Fjord, Canada. Geochimica et Cosmochimica Acta, 59, 2663-2675.
HATJE, V., PEDREIRA, R. M. A., DE REZENDE, C. E., SCHETTINI, C. A. F., DE SOUZA, G. C., MARIN, D. C. & HACKSPACHER, P. C. 2017. The environmental impacts of one of the largest tailing dam failures worldwide. Scientific Reports, 7, 10706.
HUERTA-DIAZ, M. A. & MORSE, J. W. 1990. A quantitative method for determination of trace metal concentrations in sedimentary pyrite. Marine Chemistry, 29, 119-144.
HUERTA-DIAZ, M. A. & MORSE, J. W. 1992. Pyritization of trace metals in anoxic marine sediments. Geochimica et Cosmochimica Acta, 56, 2681-2702.
JØRGENSEN, B. B., FINDLAY, A. J. & PELLERIN, A. 2019. The Biogeochemical Sulfur Cycle of Marine Sediments. Frontiers in microbiology, 10, 849-849.
KALNEJAIS, L. H., MARTIN, W. R. & BOTHNER, M. H. 2015. Porewater dynamics of silver, lead and copper in coastal sediments and implications for benthic metal fluxes. Science of The Total Environment, 517, 178-194.
KAUSHIK, A., KANSAL, A., SANTOSH, MEENA, KUMARI, S. & KAUSHIK, C. P. 2009. Heavy metal contamination of river Yamuna, Haryana, India: Assessment by Metal Enrichment Factor of the Sediments. Journal of Hazardous Materials, 164, 265-270.
KOLLURU, G. K., SHEN, X., BIR, S. C. & KEVIL, C. G. 2013. Hydrogen sulfide chemical biology: Pathophysiological roles and detection. Nitric Oxide, 35, 5-20.
LÜCKGE, A., HORSFIELD, B., LITTKE, R. & SCHEEDER, G. 2002. Organic matter preservation and sulfur uptake in sediments from the continental margin off Pakistan. Organic Geochemistry, 33, 477-488.
MARTA-ALMEIDA, M., MENDES, R., AMORIM, F. N., CIRANO, M. & DIAS, J. M. 2016. Fundão Dam collapse: Oceanic dispersion of River Doce after the greatest Brazilian environmental accident. Marine Pollution Bulletin, 112, 359-364.
MIDDELBURG, J. J. 1991. Organic carbon, sulphur, and iron in recent semi-euxinic sediments of Kau Bay, Indonesia. Geochimica et Cosmochimica Acta, 55, 815-828.
MOREIRA, M., DÍAZ, R., SANTOS, H., MENDOZA, U., BÖTTCHER, M., CAPILLA, R., ALBUQUERQUE, A. & MACHADO, W. 2018. Sedimentary trace element sinks in a tropical upwelling system. Journal of Soils and Sediments, 18.
MORSE, J. W. 1994. Interactions of trace metals with authigenic sulfide minerals: implications for their bioavailability. Marine Chemistry, 46, 1-6.
MORSE, J. W. & LUTHER, G. W. 1999. Chemical influences on trace metal-sulfide interactions in anoxic sediments. Geochimica et Cosmochimica Acta, 63, 3373-3378.
O’NEIL, J. M., DAVIS, T. W., BURFORD, M. A. & GOBLER, C. J. 2012. The rise of harmful cyanobacteria blooms: The potential roles of eutrophication and climate change. Harmful Algae, 14, 313-334.
OTERO, X. L., HUERTA-DIAZ, M. A. & MACı́AS, F. 2003. Influence of a turbidite deposit on the extent of pyritization of iron, manganese and trace metals in sediments from the Guaymas Basin, Gulf of California (Mexico). Applied Geochemistry, 18, 1149-1163.
PRAHARAJ, T. & FORTIN, D. 2004. Determination of Acid Volatile Sulfides and Chromium Reducible Sulfides in Cu-Zn and Au Mine Tailings. Water, Air, and Soil Pollution, 155, 35-50.
QUARESMA, V. S., BASTOS, A. C., LEITE, M. D., COSTA, A., CAGNIN, R. C., GRILO, C. F., ZOGHEIB, L. F. & SANTOS OLIVEIRA, K. S. 2020. The effects of a tailing dam failure on the sedimentation of the eastern Brazilian inner shelf. Continental Shelf Research, 205, 104172.
QUEIROZ, H. M., FERREIRA, T. O., BARCELLOS, D., NÓBREGA, G. N., ANTELO, J., OTERO, X. L. & BERNARDINO, A. F. 2021a. From sinks to sources: The role of Fe oxyhydroxide transformations on phosphorus dynamics in estuarine soils. Journal of Environmental Management, 278, 111575.
QUEIROZ, H. M., NÓBREGA, G. N., FERREIRA, T. O., ALMEIDA, L. S., ROMERO, T. B., SANTAELLA, S. T., BERNARDINO, A. F. & OTERO, X. L. 2018a. The Samarco mine tailing disaster: A possible time-bomb for heavy metals contamination? Science of The Total Environment, 637-638, 498-506.
QUEIROZ, H. M., NÓBREGA, G. N., OTERO, X. L. & FERREIRA, T. O. 2018b. Are acid volatile sulfides (AVS) important trace metals sinks in semi-arid mangroves? Marine Pollution Bulletin, 126, 318-322.
QUEIROZ, H. M., YING, S. C., ABERNATHY, M., BARCELLOS, D., GABRIEL, F. A., OTERO, X. L., NÓBREGA, G. N., BERNARDINO, A. F. & FERREIRA, T. O. 2021b. Manganese: The overlooked contaminant in the world largest mine tailings dam collapse. Environment International, 146, 106284.
RAISWELL, R., BUCKLEY, F., BERNER, R. & ANDERSON, T. Degree of pyritization of iron as a paleoenvironmental indicator of bottom-water oxygenation. 1988.
SANTOLIN, C. V. A., CIMINELLI, V. S. T., NASCENTES, C. C. & WINDMÖLLER, C. C. 2015. Distribution and environmental impact evaluation of metals in sediments from the Doce River Basin, Brazil. Environmental Earth Sciences, 74, 1235-1248.
TYRRELL, T. 1999. The relative influences of nitrogen and phosphorus on oceanic primary production. Nature, 400, 525-531.
VAALGAMAA, S., SONNINEN, E., KORHOLA, A. & WECKSTRÖM, K. 2013. Identifying recent sources of organic matter enrichment and eutrophication trends at coastal sites using stable nitrogen and carbon isotope ratios in sediment cores. Journal of Paleolimnology, 50.
WIJSMAN, J. W. M., MIDDELBURG, J. J., HERMAN, P. M. J., BÖTTCHER, M. E. & HEIP, C. H. R. 2001. Sulfur and iron speciation in surface sediments along the northwestern margin of the Black Sea. Marine Chemistry, 74, 261-278.
YANG, Z., WANG, Y., SHEN, Z., NIU, J. & TANG, Z. 2009. Distribution and speciation of heavy metals in sediments from the mainstream, tributaries, and lakes of the Yangtze River catchment of Wuhan, China. Journal of Hazardous Materials, 166, 1186-1194.
YE, S., LAWS, E., WU, Q., ZHONG, S., DING, X., GUANGMING, Z. & GONG, S. 2010. Pyritization of trace metals in estuarine sediments and the controlling factors: A case in Jiaojiang Estuary of Zhejiang Province, China. Environmental earth sciences, 61, 973-982.