Autores
Martínez Ramirez, A.P. (UNIVERSIDAD INDUSTRIAL DE SANTANDER) ; Blanco Tirado, C. (UNIVERSIDAD INDUSTRIAL DE SANTANDER) ; Combariza, M.Y. (UNIVERSIDAD INDUSTRIAL DE SANTANDER)
Resumo
Colombia is the fourth palm oil producer worldwide. The processing of palm oil
fruits yields abundant lignocellulosic residues such as empty fruit bunches
(EFBs) and effluents like Palm Oil Mill Effluent (POME). POME is a harmful
effluent for aquatic ecosystems due to its high pigment and organic matter
contents. We investigate the catalytic behavior of an EFBs lignocellulosic
fibers/iron oxide biocomposite as an advanced oxidation process (AOP) for POME
treatment. FXD, XRD, TGA, and SEM measurements showed the amount of iron
deposited on the EFB fibers, its thermal behavior, and the nanoparticle
distribution at the surface. The bionanocomposites reduced color in POME by 90 %
and COD by 55 %.
Palavras chaves
Empty fruit bunch (EFB); palm oil mill effluent; iron oxide nanoparticles
Introdução
Untreated industrial wastewater discharges cause adverse environmental impacts
worldwide (Unidas., 2017.) Searching for efficient yet inexpensive processes for
industrial effluent treatment is vital to mitigate water pollution. Colombia is
an agricultural country and the world's fourth largest palm oil producer (Mesa &
Azuero, 2021). High amounts of aqueous and solid wastes result from palm oil
extraction. About five kilograms of POME, the aqueous residue, are produced per
kilogram of palm oil (Lam & Lee, 2011). POME is a complex effluent defined as an
acidic aqueous colloidal suspension of cellulosic residues, oil, suspended
solids, and natural dyes (Althausen, 2016). Untreated POME discharges to surface
waters are harmful to delicate tropical ecosystems, the regions of the world
where Palm oil is mainly grown. POME hampers aquatic autotrophs' photosynthesis
due to its high organic load and color (Kim, 2021).
POME is a highly complex effluent equally challenging to treat, with a chemical
oxygen demand (COD) ranging from 15,000 to 100,000 mg/L, biochemical oxygen
demand (BOD) around 42,000 mg/L, a pH between 3.5 and 4.2, and a temperature
between 80-90°C (Yashni, Al-Gheethi, Radin Mohamed, Arifin, & Mohd Salleh,
2020). Current POME treatments involve storage ponds where the organic matter
slowly decomposes microbiologically into methane (CH4) and carbon dioxide (CO2).
Suspended solids settle out during this process, and the resultant sludge
requires further treatment (Cheng et al., 2021; Enström et al., 2019). However,
this treatment does not entirely eliminate COD and does not remove color, making
POME very harmful to the aquatic ecosystem (Sani et al., 2020).
Advanced oxidation processes (AOPs) for aqueous effluent treatment are at the
vanguard of alternatives for recalcitrant pollutants removal. In AOPs, a strong
oxidizing agent (e.g., the hydroxyl radical) is used to degrade pollutants
(Miklos et al., 2018). The homogeneous Fenton reaction ranks amongst the most
cost-efficient AOPs. In this process, a mixture of ferrous ions and H2O2 under
acidic conditions produces highly reactive species such as hydroxyl radicals
(Sani et al., 2020; Yashni et al., 2020). However, the homogeneous Fenton
process results in sludge formation (mainly containing iron (III) complexes),
making catalyst recovery and recyclability difficult (Sani et al., 2020a). The
heterogeneous Fenton process can be an alternative to overcome these drawbacks.
We have previously reported Fenton-like heterogeneous processes where the
catalyst is supported on lignocellulosic matrices (Ortiz et al., 2014; Ravelo,
2013). Using solid supports allows the catalyst to be recovered and reused. The
inherent surface rugosity and porosity of lignocellulosic fibers lend themselves
to promoting in-situ deposition of reactive nanoparticles. In addition, these
materials have a large surface area, thermal stability, and biodegradability
(Nasrollahzadeh et al., 2021). Palm oil extraction produces abundant residual
lignocellulosic biomass, such as empty fruit bunches (EFBs). Increasing
materials circularity in agro-industrial processes helps reduce costs and
environmental impacts. We explore using residual EFB as a lignocellulosic matrix
for iron-oxide in situ synthesis. The resultant biocomposite was tested as
functional material in a heterogeneous Fenton-like process to remove COD and
color from POME. EFB/FexOy biocomposites showed efficiencies up to 90% for color
and 50% for COD removal from POME samples.
Material e métodos
Alkaline hydrogen peroxide (AHP) treatment:
EFB fibers were immersed in an alkaline H2O2 solution (pH 11.5). The
mixture was
allowed to react for 2 hours at 70°C. Subsequently, the EFB fibers were washed
and dried. The delignified EFB sample was labeled EFB-AHP.
NaOH and HCl treatment:
EFB fibers were placed in 200 ml of a NaOH solution (5% wt) and left to soak for
one hour. Then, the EFB sample was transferred to 200 ml of an HCl solution (5 %
wt) and left to react for one hour. Afterward, the fibers were washed and dried.
The NaOH/HCl treated samples were labeled EFB-NaOH-HCl.
In-situ deposition of iron oxides nanoparticles:
EFB, EFB-AHP, and EFB-NaOH-HCl samples were immersed in 200 mL of a Fe2+:Fe3+
(1:1.2) solution for 10 minutes. Then, ammonia was added dropwise until pH 6.
The mixture was left to stand for 1 hour, and the fibers were washed and dried.
The resultant biocomposites were labeled EFB-Fe, FB-AHP-Fe, and EFB-NaOH-HCl-Fe.
Color and COD removal:
A POME sample was placed in contact with a biocomposite sample, and H2O2 was
added to the mixture. The influence of POME:biocomposite ratio and the
temperature were tested for the removal of color and COD.
Measurements:
XRD, XRF, SEM, SEM-EDS and TGA were used to characterize the EFB, FB-AHP, EFB-
NaOH-HCl, EFB-Fe, FB-AHP-Fe, and EFB-NaOH-HCl-Fe samples. POME color before and
after treatment was determined at 3 wavelengths according to the ISO 7887 of
2011 method B. COD was measured according to the SM 5200 ed 22/2012. Triplicate
color and COD measurements were performed.
Resultado e discussão
Characterization of the matrices:
The AHP and NaOH/HCl treatments introduced morphological changes to the EFBs
surfaces, as shown in Figure 1. Interestingly, raw EFB fibers exhibit well-
defined micrometric-sized pores, some filled with SiO2 particles (Figure 1a). In
the palm oil plant, the SiO2 originates from soil minerals (Khalil et al., 2008;
Wong et al., 2020) and is deposited into the pores within the cell wall to
increase the resistance of the fiber's outer protective layers (Yunus et al.,
2010). After EFB fibers pretreatment, part or all the SiO2 in the surface is
removed. The NaOH-HCl treatment removes all the SiO2 present and delivers
smoother and more porous surfaces than the AHP-treated samples (Figures 1 b and
1c). Finally, none of the treatments affected the fiber's integrity.
The compositional content of EFB, AHP and EFB-NaOH-HCl was determined by X-ray
fluorescence (XRF), as shown in Table 1. The amount of SiO2decreased with the
AHP and NaOH/HCl treatment; corroborating that SiO2 is indeed in the internal
cavities of the fibers (Yunus et al., 2010). Also, the amount of elements such
as potassium, chlorine, calcium, magnesium, sulfur, phosphorus, aluminum, and
iron were decreased/removed. The presence of sodium is associated with using
NaOH in the pretreatments.
Characterization of Biocomposites:
Electron micrographs show the presence of iron NPs in the EFB fibers (Figure 2).
We found that NPs coat the silicon oxides present in the fibers. At the same
time, a better distribution of the NPs was observed in the EFB fibers than in
the other fibers. This was associated with the surface changes due to the
delignification and NaOH-HCl treatment.
The results obtained by XRF are presented in Table 2. The amount of iron varied
in each bionanocomposite. 1.8, 2.54 and 3.0 % of Fe2O3 were obtained for EFB-Fe,
AHP-Fe and EFB-NaOH-HCl-Fe respectively. AHP and NaOH/HCl pretreatments allow
for a better in-situ deposition of iron oxide NPs. In this case, we expect the
removal of color and COD from POME to be higher using EFB treated with NaOH and
HCl.
Color and COD removal:
Table 3 shows the percentage color at 3 wavelengths and COD removal of the
treated POME. We expected that biocomposite (R-NaOH-HCl-Fe) with a higher amount
of iron oxides would remove a higher amount of color and COD. But EFB-Fe was the
material that removed the greatest amount of color (96.2 %) and COD (64.1 %).
However, the biocomposites showed removals of the amount of color and COD
greater than 92% and 55%, respectively. Researches have reported removal of 92.1
% of color and 85.1 % (Saeed et al., 2015) and 63 % of COD using homogeneous
Fenton. For heterogeneous Fenton, the COD removal amount was higher than 92 %
(Sani et al., 2020b). Our results are like those reported. However, it is
important to note that we treated the effluent with solid wastes, both from oil
palm processing and oil palm processing.
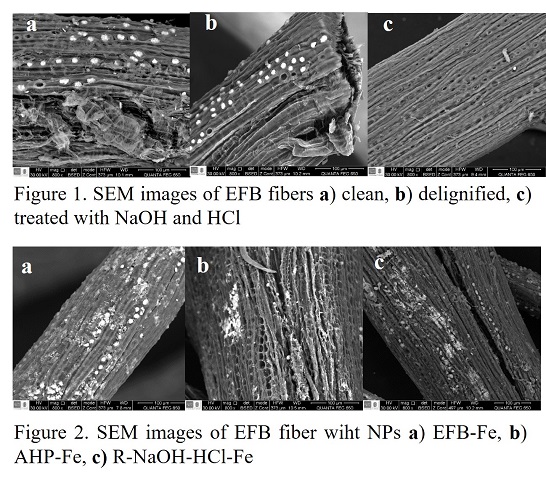
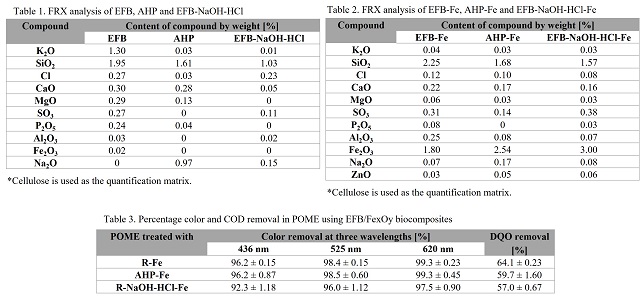
Conclusões
SEM and XRF characterization techniques indicated the removal of surface
impurities by using delignification and treatment with NaOH and HCl. Likewise,
they corroborated the presence of iron oxide NPs. The amount of iron oxide NPs in
biocomposites by XRF was 3.00 % in EFB-NaOH-HCl-Fe, 2.54 % in AHP-Fe and 1.80 % in
R-Fe. The amount of NPs in the untreated fiber was lower. However, it was observed
that R-Fe removed a higher amount of color (96 %) and COD (64 %) than the other
biocomposites. But the percentage removal of color and COD of the biocomposites
are close. Therefore, it is not necessary to perform a delignification process or
treatment with NaOH-HCl to increase the removal efficiency.
Agradecimentos
We thank the Guatiguará Technology Park at Universidad Industrial de Santander for
infrastructural support. Andrea Martínez acknowledges Universidad Industrial de
Santander for a graduate scholarship.
Referências
Cheng, Y. W., Chong, C. C., Lam, M. K., Ayoub, M., Cheng, C. K., Lim, J. W., Yusup, S., Tang, Y., & Bai, J. (2021). Holistic process evaluation of non-conventional palm oil mill effluent (POME) treatment technologies: A conceptual and comparative review. Journal of Hazardous Materials, 409(October 2020), 124964. https://doi.org/10.1016/j.jhazmat.2020.124964
Enström et al. (2019). Introducing a new GHG emission calculation approach for alternative methane reduction measures in the wastewater treatment of a palm oil mill. Environment, Development and Sustainability, 21(6), 3065–3076. https://doi.org/10.1007/s10668-018-0181-4
Khalil, H. P. S. A., Alwani, M. S., Ridzuan, R., Kamarudin, H., & Khairul, A. (2008). Chemical composition, morphological characteristics, and cell wall structure of Malaysian oil palm fibers. Polymer - Plastics Technology and Engineering, 47(3), 273–280. https://doi.org/10.1080/03602550701866840
Kim. (2021). Adoption of TiO2-photocatalysis for palm oil mill effluent (POME) treatment: Strengths, weaknesses, opportunities, threats (SWOT) and its practicality against traditional treatment in Malaysia. Chemosphere, 270, 129378. https://doi.org/10.1016/j.chemosphere.2020.129378
Lam, M. K., & Lee, K. T. (2011). Renewable and sustainable bioenergies production from palm oil mill effluent (POME): Win-win strategies toward better environmental protection. In Biotechnology Advances (Vol. 29, Issue 1, pp. 124–141). Elsevier Inc. https://doi.org/10.1016/j.biotechadv.2010.10.001
Mesa Dishington, J., & Azuero Garcia, A. F. (2021). Balance 2020 y perspectivas 2021 de la agroindustria de la palma de aceite. Fedepalma, 41.
Miklos, D. B., Remy, C., Jekel, M., Linden, K. G., Drewes, J. E., & Hübner, U. (2018). Evaluation of advanced oxidation processes for water and wastewater treatment – A critical review. Water Research, 139, 118–131. https://doi.org/10.1016/j.watres.2018.03.042
Nasrollahzadeh, M., Soleimani, F., Bidgoli, N. S. S., Nezafat, Z., Orooji, Y., & Baran, T. (2021). Recent developments in polymer-supported ruthenium nanoparticles/complexes for oxidation reactions. Journal of Organometallic Chemistry, 933, 121658. https://doi.org/10.1016/j.jorganchem.2020.121658
Ortiz, P., Ovalle, S., Blanco-Tirado, C., & Combariza, M. (2014). Síntesis in situ de nanopartículas de óxidos de hierro soportadas en fibras de fique y su uso en la degradación de colorantes. MATERIALES COMPUESTOS Y RECUBRIMIENTOS, 2, 152–158.
Ravelo, E. (2013). Síntesis y caracterización de bionanocompositos con base en fibras de fique y óxido de hierro (Fe3O4), y evaluación de su actividad en la degradación de colorantes. [Tesis de pregrado, Universidad Industrial de Santander]. http://tangara.uis.edu.co/biblioweb/pags/cat/popup/pa_detalle_matbib.jsp?parametros=166889|%20|12|25
Saeed, M. O., Azizli, K., Isa, M. H., & Bashir, M. J. K. (2015). Application of CCD in RSM to obtain optimize treatment of POME using Fenton oxidation process. Journal of Water Process Engineering, 8, e7–e16. https://doi.org/10.1016/j.jwpe.2014.11.001
Sani, S., Dashti, A. F., & Adnan, R. (2020a). Applications of Fenton oxidation processes for decontamination of palm oil mill effluent: A review. Arabian Journal of Chemistry, 13(10), 7302–7323. https://doi.org/10.1016/j.arabjc.2020.08.009
Sani, S., Dashti, A. F., & Adnan, R. (2020b). Applications of Fenton oxidation processes for decontamination of palm oil mill effluent: A review. In Arabian Journal of Chemistry (Vol. 13, Issue 10, pp. 7302–7323). Elsevier B.V. https://doi.org/10.1016/j.arabjc.2020.08.009
Unidas., N. (2017). Agua | Naciones Unidas. Retrieved September 8, 2019, from https://www.un.org/es/sections/issues-depth/water/index.html
Wong, W. Y., Lim, S., Pang, Y. L., Shuit, S. H., Chen, W. H., & Lee, K. T. (2020). Synthesis of renewable heterogeneous acid catalyst from oil palm empty fruit bunch for glycerol-free biodiesel production. Science of the Total Environment, 727. https://doi.org/10.1016/j.scitotenv.2020.138534
Yashni, G., Al-Gheethi, A., Radin Mohamed, R. M. S., Arifin, S. N. H., & Mohd Salleh, S. N. A. (2020). Conventional and advanced treatment technologies for palm oil mill effluents: a systematic literature review. Journal of Dispersion Science and Technology, 0(0), 1–19. https://doi.org/10.1080/01932691.2020.1788950
Yunus, R., Salleh, S. F., Abdullah, N., & Biak, D. R. A. (2010). Effect of ultrasonic pre-treatment on low temperature acid hydrolysis of oil palm empty fruit bunch. Bioresource Technology, 101(24), 9792–9796. https://doi.org/10.1016/j.biortech.2010.07.074