Autores
Segura, R. (UNIVERSIDAD DE VALPARAISO) ; Peón, F. (UNIVERSIDAD DE VALPARAISO) ; Olivares, F. (UNIVERSIDAD TECNICA FEDERICO SANTA MARIA) ; Henriquez, R. (UNIVERSIDAD TECNICA FEDERICO SANTA MARIA) ; Hevia, S. (PONTIFICIA UNIVERSIDAD CATÓLICA DE CHILE)
Resumo
Hybrid films composed by TiO2 and functionalized MWCNTs were synthesized and used
as photoanodes to perform the water splitting process under neutral and free of
sacrificial agents medium. The results show that introducing a sublayer of MWCNTs
functionalized with –NO2 and –COOH groups leads to structural modifications on
TiO2 that decrease the band gap and reduce the recombination phenomenon. Those
groups anchored to nanotubes also play an important role in their dispersibility
and provide an additional electron withdrawing effect to the pristine nanotubes.
These conditions significantly improve the photoconversion efficiency of the
hybrid system until 15-fold regarding pristine TiO2 prepared under the same
conditions.
Palavras chaves
Photoanode; Photoelectrocatalyst; Water splitting
Introdução
Several strategies have been developed to improve the photocatalytic efficiency
of TiO2 [1]. These strategies seek to extend the optical absorption of
photoelectrocatalytic devices to the visible range and/or reduce the
recombination of the photogenerated electron/hole pairs. A large number of
studies have been developed that combine this semiconductor with carbon
nanomaterials such as graphene [2] and carbon nanotubes (CNTs) [3,4]. CNTs are
known for their high electrical conductivity, together with the large surface-
volume ratio, make them an ideal material for charge transport of the electrons
collected by the photoactive material. However, the low solubility of this
material in most common solvents limits its use. To reduce this limitation, it
is convenient to chemically modify the CNTs, functionalizing them before
combining them with the semiconductor. Recently, a theoretical study has shown
that in CNTs functionalized with nitro and carboxyl groups, the electron density
of these groups shifts toward the nanotube, which increases the number of charge
carriers in the nanotube, leading to improved electrical conductivity [5]. In
this work we report the chemical vapor deposition (CVD) synthesis of hybrid
films of TiO2 and MWCNTs functionalized with nitro (-NO2) and carboxyl (-COOH)
groups on a silicon substrate with the purpose of improving the photocatalytic
efficiency of TiO2 in the water splitting reaction.
Material e métodos
MWCNTs were synthesized via CVD [6] and then treated with a concentrated
solution of H2SO4/HNO3 acids in a 10/9 v/v ratio at 60°Cx90min to favor the
nitro functionalization instead of pure carboxyl groups [7]. Those samples were
labeled as “nox-MWCNTs”. TiO2 films were grown by CVD using Titanium (IV)
tetraisopropoxide [8] as precursor (500°Cx30 min). The TiO2 films were deposited
on Si(100) and nox-MWCNTs/Si(100). The materials were characterized by TEM, SEM,
XRD, Raman, FTIR, and DRS-UV-vis. The photoelectrochemical response was
evaluated using an EZstat-Pro potentiostat and a Newport-Oriel solar simulator
(150 W Xe-lamp). Measurements were made in 0.1M KCl using a Pt wire counter-
electrode, Ag/AgCl reference-electrode, and the samples as working-electrode.
Resultado e discussão
Figure 1(a,b) show a SEM image of the grainy morphology of TiO2 film grown
directly on Si(100), which exhibit an average thickness of ~350 nm. Fig.1(b)
presents a SEM image of nox-MWCNTs film and Fig.1(d) a TEM image. Fig.1(e) shows
the TiO2/nox-MWCNTs/Si(100) composite. Unlike the TiO2 film grown on bare Si,
the film grown on nox-MWCNTs forms a thinner layer that covers the nanotubes so
that it is still possible to view their morphology.
The photoelectrochemical characterization of the pure and modified TiO2 was
performed using LSV (Fig.2(a)). In all cases, the current density rise with the
voltage, and its value in dark is minima compared with the response under
illumination. We can also observe much higher photocurrent density values for
the modified systems compared with pure TiO2. By example, the current density
values for TiO2/Si, TiO2/MWCNTs/Si and TiO2/nox-MWCNTs/Si under lighting at 1 V
are around 0.3, 1.0 and 2.6 mA/cm2 respectively. Fig. 2(b) showed the curves of
transient photocurrent response.
Fig.2(d) shows the graphs of photoconversion efficiency (ABPE) for all systems.
For the TiO2/Si system, the efficiency increases with the applied voltage and we
can observe two maximums, one close to 0.4-0.5 V (~0.023%), which looks like a
shoulder, and another close to 0.9V (~0.035%). The photoanode modified with
pristine MWCNTs has a maximum at 0.55 V with a photocurrent ~0.17 mA/cm2 and
~0.11% of efficiency. On the other hand, the TiO2/nox-MWCNTs/Si system has a
maximum at 0.57 V with a photocurrent ~0.44 mA/cm2 and ~0.3% efficiency. In both
modified systems there was a significant improvement of photoconversion
efficiency close to 4.6 and 12.5 times greater than pure TiO2[7].
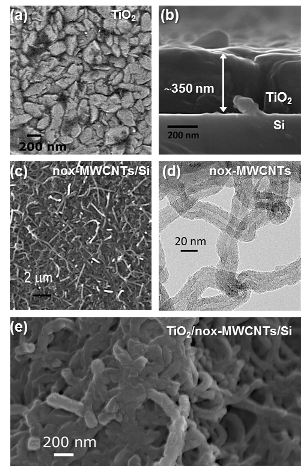
(a, b) SEM of TiO2 film grown by CVD on Si(100). (c) SEM, and (d) TEM image of nox-MWCNTs. (g) SEM image of TiO2/nox-MWCNTs/Si(100).
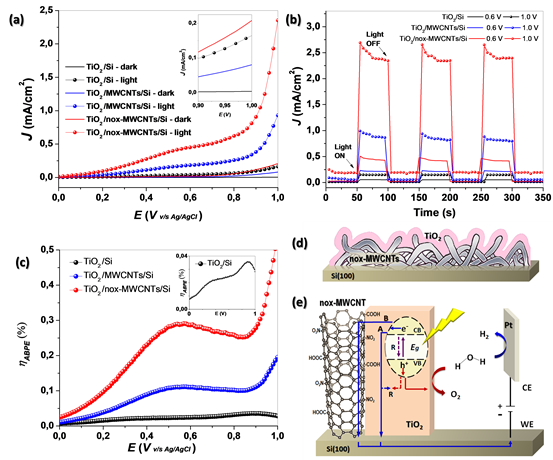
(a) LSV, (b) transient photocurrent response at 0.6 V and 1.0 V, and (c) ABPE curves for the TiO2/Si, TiO2/MWCNTs/Si and for TiO2/nox-MWCNTs/Si system
Conclusões
As was found, the combination of TiO2 with MWCNTs functionalized with –NO2 and –
COOH increases the number of charge carriers of the nanotube leading to improved
electrical conductivity, suppression of recombination process, and also a
narrowing of band gap. We have shown that MWCNTs functionalized with a mix of
nitro and carboxyl groups constitute an outstanding material for the construction
of a TiO2-based photoanode, significantly improving the efficiency in the water
splitting process compared with the pure semiconductor.
Agradecimentos
This research is funded by Fondecyt #1161614 and #1181905 grants, ANID Doctoral
Fellowship N°21200362 (F.Peón).
Referências
[1] A. Fujishima, T.N. Rao, D.A. Tryk, J. Photochem. Photobiol. C Photochem. Rev. 1 (2000) 1–21.
[2] X. Zhang, Y. Sun, X. Cui, Z. Jiang, Int. J. Hydrogen Energy 37 (2012) 811–815.
[3] P. Soto, F. Olivares, S. Gómez, G. Cabrera, R. Villalonga, R. Segura, Thin Solid Films 656(2018) 30-36.
[4] A.M. Ahmed, F. Mohamed, A.M. Ashraf, M. Shaban, A. Parwaz, A.M. Asiri, Chemosphere 238 (2020) 124554.
[5] N.P. Boroznina, I.V. Zaporotskova, Russ. Microelectron. 46(2017) 580–584.
[6] R. Segura, G. Cardenas, P. Häberle, Phys. Stat. Sol.(a) 204 (2007) 513–517.
[7] F. Olivares, R. Segura, J. Reyes, F. Peón, R. Henríquez, S. Hevia, B. Durán, R. Villalonga, Materials Letters 2021, 285, 129128.