Autores
González, R. (UNIVERSIDAD DE TALCA) ; Prent Peñalosa, L. (UNIVERSIDAD ANDRES BELLO) ; Gutierrez, M. (UNIVERSIDAD DE TALCA) ; Caballero, J. (UNIVERSIDAD DE TALCA) ; F. de La Torre, A. (UNIVERSIDAD DE CONCEPCIÓN)
Resumo
Some new N–benzyl–2–(N–benzylamido)acetamide (NBNBA) peptoids were successfully
synthesized by Ugi four–component reactions and they were evaluated as
acetylcholinesterase (AChE) and butyrylcholinesterase (BChE) inhibitors. Compounds
5a and 5d showed high selectivity for butyrylcholinesterase with values of IC50 of
28 and 40 μM, respectively. Lineweaver–Burk plot analysis showed that active
compounds were competitive inhibitors. In addition, molecular docking studies were
performed to propose the molecular interactions and binding modes of 5a and 5d in
the active sites of AChE and BChE. The models allow explaining the selectivity for
BChE. Finally, pharmacokinetic parameters were calculated, and good
biodisponibility and low toxicity were predicted for the studied compounds.
Palavras chaves
cholinesterase inhibitors; peptoids; multicomponent reactions
Introdução
Alzheimerʹs disease (AD) is a chronic, progressive, fatal, and the most common neurodegenerative disease, due to its prevalence in recent years, it has become a priority issue relevant to public health (World Alzheimer Report 2021). AD is clinically characterized by progressive memory loss, a decline in language skills, and progressive deficits in different cognitive domains (Song, 2018). The therapeutic options for AD treatment are limited to three approved acetylcholinesterase (AChE) inhibitors: donepezil, rivastigmine, and galantamine. However, these commercial drugs for AD treatment cannot meet the market demand and do not reverse the succession of AD (KUSHWAHA, 2019). Thus, it is necessary to develop novel anti–AD drugs that can act as far upstream as possible in the neurodegenerative cascade. Peptoids are compounds structurally similar to peptides, which can be synthesized without major complications and are stable; therefore, they have been considered in drug–discovery processes for several decades. The structural differences they have with peptides, allows them to have properties such as high resistance to proteolytic activity and generally improved pharmacokinetics (ZUCKERMANN, 2011; LAMBRUSCHINI, 2017). These compounds are included among the categories of peptidomimetics.
Peptoids have been reported as potential agents against AD. For instance, Liu et al. (Liu, 2017) described the non-toxic and non–fibrogenic peptoid CPO_Aβ17–21P, which generates a decrease in the apolipoprotein E4(apoE4)/amyloid–beta (Aβ) and attenuates the prophylogenic effects of apoE4 on Aβ aggregation in vitro as well as apoE4 potentiation of Aβ cytotoxicity. In this sense, this peptoid has significant promise as a new therapeutic agent for AD. In another report, Luo et al. (LOU, 2013) synthesized the peptoid IAM1, which binds selectively to Aβ42 and inhibited Aβ42 aggregation in vitro. In other work, Pradhan et al. (PRADHAN, 2018) reported a peptoid that inhibits Aβ fibril formation and confers significant neuroprotection against nerve growth factor (NGF) deprived toxicity in PC12 derived neurons; this peptoid also inhibits the acetylcholinesterase (AChE). The design of peptoids as cholinesterase inhibitors has not been commonly explored. Beyond the previous example, our group recently reported the synthesis of diN–substituted glycyl–phenylalanine peptoids by using isocyanide–based multicomponent reactions (IMCRs), specifically Ugi four component reactions (Ugi–4C), and their activities as AChE and butyrylcholinesterase (BChE) inhibitors were evaluated (PRENT-PEÑALOSA, 2019).
The relevance of cholinesterases (AChE and BChE) for AD is well documented in the literature. The family of cholinesterase enzymes plays an essential role in acetylcholine regulation and cholinergic signaling (MESULAM, 2002). AChE is highly expressed in the brain and is substrate-specific, while BChE can be found in the whole body and is non–specific. Both enzymes are highly efficient in the degradation of the neurotransmitter ACh, degrading it at a swift rate (up to 10,000 molecules per second). Both enzymes display different kinetic characteristics depending on ACh concentrations. AChEʹs activity becomes highly dominant at low ACh concentrations, while BChE is more efficient in hydrolysis at high neurotransmitter concentrations (MUSHTAQ, 2014; ARYA, 2021). There is a significant prevalence of the design of AChE inhibitors in literature but it has been recognized that selective BChE inhibition could also be interesting for AD treatment.
Using Ugi–4C synthetic methodology we were able to prepare a series six new N–benzyl–2–N–benzylamido)acetamide (NBNBA) peptoids and they were purified and characterized, with yields more significant than 50%. the compounds were evaluated by Ellman's procedure to check their potential inhibitory activities against AChE and BChE. Interestingly, our compounds were highly selective for BChE. Molecular docking analyses were done to explain such selectivity.
Material e métodos
Reagents were purchased from Merck (Germany), J.T. Baker (USA), and Sigma and Aldrich Chemical Co. (USA), and used without further purification. The reaction progress was monitored using thin–layer chromatography on PF254 aluminum sheets. Column chromatography was performed using Silica gel (60–120 mesh), and solvents employed were of analytical grade. The uncorrected melting points were determined using a Fisher–Johns melting point apparatus. ATR–FTIR spectra were recorded by using UATR two. MS analyses were conducted on a Bruker Compact QqTOF. NMR spectra (1H and 13C) were measured on a Bruker Ultrashield–400 spectrometer (400 MHz 1H NMR and 100 MHz 13C NMR), using deuterated chloroform as solvent and TMS as reference.
Ugi–4CR procedure (MARCACCINI, 2007): to a solution of the amine (0.5 mmol) and the aldehyde (0.5 mmol) was added the carboxylic acid component (0.5 mmol) and the isocyanide (0.5 mmol) in MeOH (2.5 mL) and were stirred at 25 °C for 24 h. The reaction was stopped by washing once with acid citric saturated solution (25 mL), and then using sodium bicarbonate saturated solution (25 mL) and finally brine (25 mL), to remove starting substrates. The solution was dried over anhydrous sodium sulfate. The volatiles were rotoevaporated and the crude was purified by flash column chromatography to obtain the NBNBA peptoids.
Inhibitory activities of cholinesterases were analyzed using Ellmanʹs colorimetric method (ELLMAN, 1961). The samples were distributed in 96–well plates (50 μL) dissolved in phosphate buffer. An enzymatic solution of AChE/BChE (50 μL, 0.20 unit/mL) from Electrophorus electricus and equine serum was added respectively. The sample and enzyme solutions were pre-incubated for 30 min at room temperature. Then, 100 μL the substrate solution, composed of Na2HPO4 (40 mM), acetylthiocholine/butyrylthiocholine (0.24 mM), and 5,5–dithio–bis(2–nitrobenzoic acid) (0.2 mM, Ellman’s reagent), was added to all. The absorbance was measured at 405 nm after 5 min. AChE/BChE inhibition was determined for each compound. The enzymatic activity was calculated as a percentage and compared with the control. The peptoids were tested in dilution intervals ranging from 500 to 0.98 μg/mL. Each assay was run in triplicate, galantamine was used as reference. The IC50 values were determined using regression analysis. The kinetic studies of the most active compounds (5a and 5d) were performed according to the Ellmanʹs method.
ADME predictions were carried out using the web tool SwissADME and ProTox–II platform was used for toxicity predictions (DAINA, 2017; BANERJEE, 2018). Molecular docking analysis was performed to describe the interactions between compounds and enzymes (AChE, BChE). Cholinesterase structures were downloaded from the Protein Data Bank website (AChE– (–)–Huprine with PDB code 1E66 and BChE–Tacrine with PDB code 4BDS), and they were prepared using the Protein Preparation Wizard in Maestro (Schrödinger, LLC: USA) (GREENWOOD, 2010).
Resultado e discussão
This work aimed to synthesize NBNBA peptoids, and then they were tested as AChE and BChE inhibitors. Structurally, the peptoids were designed with selected molecular fragments that, in turn, would give the molecules the possibility of having pharmacophores that were capable of interacting with the cholinesterase targets. Various studies carried out previously have shown compounds that contain condensed aromatic cores as molecular fragments that contain heteroatoms such as nitrogen or functions such as cinnamates, benzoates and benzyl acetates, showed potential activities both in vitro and in vivo and high efficiency as a ligand, as well as the investigations carried out using hybrids of existing drugs with coumarin and quinoline. These being compounds that have shown promising cholinesterases inhibitory activities (DE SOUZA, 2016; MARUCCI, 2021). Thus, in the isocyanide based multicomponent reaction (i.e., the Ugi–4C), to obtain the main core for NBNBA peptoids, we kept invariable the amine (Bn‒NH2), the oxo (CH2O), and the isocyanide (Bn‒NC) components (Fig. 1). Furthermore, the acid component was the only variable (R‒COOH), including some electron–rich aromatic substituents and access to the novel NBNBA peptoids 5a–5f.
We decided to obtain benzyl isocyanide with minor modifications as reported in the literature (PATIL, 2020), which gave us a yield above 80%. Once the benzyl isocyanide was synthesized, we moved our efforts to carry out the Ugi–4C, varying the acid component and keeping benzylamine and p–formaldehyde. Coumarin–3–carboxylic acid (5a), benzoic acid (5b), trans–cinnamic acid (5c), 3–quinolinic acid (5d), 3–(4–chlorophenyl) propanoic acid (5e), and p-hydroxybenzoic acid (5f) produce the desired NBNBA peptoids in good yield without any sustainable difference. Natural heterocyclic structures (compounds 5a and 5d) were included in a one–pot manner. The NBNBA peptoids were characterized using different spectroscopic and spectrometric techniques. In the FT–IR spectra, the signals corresponding to the new bonds formed and the characteristic bands of the fragments incorporated into the peptoids skeleton allow us to identify the formed compounds (stretching vibrations of the N–H bond at frequency values between 3313 and 3245 cm-1; medium intensity bands associated with the stretching vibrations of the CH–bonds, both for sp2 and sp3 carbons at frequency values between 3065–2910 cm-1 and, at frequencies around 1650 cm-1, the presence of the 2 bands of high intensity of the valence vibrations of the C = O bonds of the amides formed or of one of great intensity that probably belongs to both carbonyl groups overlapping). In the 1H NMR spectrum of compound 5a, the assignment could be made starting from the most unshielded proton attached to the carbon of the double bond of the ring of coumarin motif. This proton can be seen at a chemical shift value of 7.84 ppm in the form of a singlet. Another characteristic signal that allows us to confirm the peptoid formation is the presence of 3H at 4.41ppm and 1H at 4.07 ppm corresponding to CH2 groups. The 13C NMR spectrum shows the signals of the 3 carbonyls functional groups at 167, 165, and 159 ppm. Besides, the peaks at 143 and 118 ppm are attributable to the C at double bonds of the coumarin skeleton.
All compounds were evaluated as potential cholinesterase inhibitors (AChE and BChE) using the colorimetric Ellmanʹs methods adapted to 96–well plates. The new synthetics peptoids 5a–5f showed moderate activity as ChE inhibitors, but the compounds 5a and 5d showed high selectivity for BChE (Fig. 1). This result, together with its comparison with synthetic homologues but using cyclohexyl isocyanide, which did not show significant activities or selectivity for cholinesterases, could enhance the potential of including aromatic substituents to increase the ability to inhibit these enzymes. In addition, several researcher works have demonstrated the importance of BChE within the nervous system to be pivotal in the late stages of AD (GREIG, 2001; DARVESH, 2016). However, due to the selectivity of our NBNBA peptoids for BChE, the scaffold of these compounds could be used for further development of improved BChE selective inhibitors. Compounds 5a and 5d simultaneously had the lowest IC50 values (28 and 40 μM), for the inhibition of BChE, which could justify the condensed aromatic ring interactions with the different active fragments of the protein. The activities were moderate, but they explain the convenience of using the Ugi–4C reaction to obtain a library of biologically active compounds rapidly. Interestingly, compounds 5a and 5d are the only ones with fused rings as substituents, suggesting that the increase of aromatic interactions is better for establishing chemical interactions in the BChE binding site and for improving selectivity for this cholinesterase. An enzyme kinetic study to establish a possible inhibition mechanism were evaluated independently. The obtained Michaelis–Menten parameters and the Lineweaver–Burk plots allowed us to conclude that compound 5a would display a competitive type inhibition against BChE with variation in Km values. Compound 5a showed Km values of 34.48; 40.61 and 40.51 μM, and Vmax value of 1.20 mol/min. The compound 5d with a competitive type of inhibition against BChE with variation in values of Km and Vmax. The values of Km obtained are 26.74; 34.60 and 49.50 μM, and Vmax value of 1.20 mol/min.
Some selected molecular properties were predicted using SwissADME, a web tool that predicts the oral bioavailability of potential lead or drug molecules. The results indicated that the studied compounds had drug–like candidate properties with no violation of the drug–likeness rules, according to Lipinskiʹs rule of five. Their molecular weights were less than 500; thus, these molecules were anticipated to be easily transported, diffused, and absorbed. Both the number of hydrogen bond acceptors (O and N atoms) and the number of hydrogen bond donors (NH and OH) in compounds were also following the Lipinskiʹs rules (i.e., less than 10 and 5, respectively). Topological polar surface area (TPSA) is a utility parameter for predicting drug transport properties and shown to correlate very well with human intestinal absorption, in vitro monolayer permeability, and blood–brain barrier (BBB) penetration. NBNBA peptoids had TPSA values in order of 50–80 Å, below the limit of 160 Å. We can conclude that the evaluated compounds possess good properties as potential drugs from the results. The studied peptoids show physicochemical properties concordant with what is required by Lipinski’s rule of 5, to predict the bioavailability of potential drugs candidates (LIPINSKI, 2012). Potential toxicities were investigated using the ProTox-II webserver. The oral toxicity value for NBNBA peptoids 5a and 5d, had LD50 values, which are classified in class IV (harmful if swallowed (300<LD50≤2000)). These results conclude that the investigated compounds might be less toxic to organisms than approved drugs, although experimental in vitro/in vivo studies are necessary for supporting this conclusion.
Molecular docking experiments show that NBNBA peptoids 5a and 5d have different orientations inside AChE and BChE binding sites. AChE and BChE binding site residues were analyzed to explain why these compounds select BChE. It was noted that the bulky phenyl side chain group of Phe330 in AChE is replaced by a methyl group of Ala328 in BChE. In AChE, Phe330 is placed near the residue Trp84, forming a cavity occupied by coumarin and quinoline groups from compounds 5a and 5d, respectively. These groups are stabilized by sandwich π–π stacking interactions, similar to those observed for HU in PDB. BChE has a wider cavity due to the replacement of Phe by Ala328. Therefore, compounds 5a and 5d adopted different orientations to interact through π–π stacking with Tyr332, Phe329, Trp231, and Trp82 (Fig. 2).
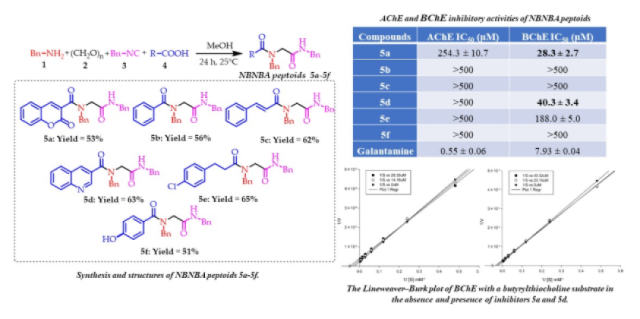
Ugi-4C synthesis and cholinesterase inhibitory results of NBNBA peptoids 5a-5f
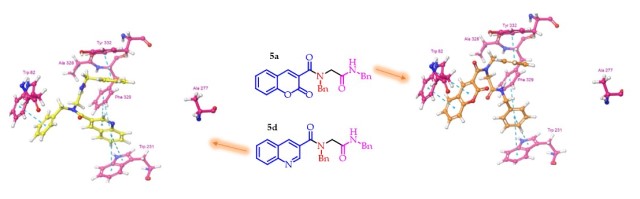
Molecular docking models for the peptoids 5a and 5d inside the BChE binding sites.
Conclusões
We report the synthesis and characterization of six new NBNBA peptoids in yields more significant than 50%. The structures were elucidated using conventional spectroscopy and spectrometry techniques. The obtained compounds were tested as AChE and BChE inhibitors, showing moderate inhibition values, and compounds 5a and 5d had good selectivity for BChE. The kinetic study showed a competitive action mode of the most active compounds, and molecular docking showed important interactions between the compounds and BChE, reinforcing the selective behavior observed in the in vitro analysis. According to theoretical calculations, the NBNBA peptoids presented good ADMET properties, which is a good indication that these molecules could potentially apply to the pharmacological field. Docking calculations revealed that compounds 5a and 5d are stabilized inside the BChE binding site through π–π stacking with the aromatic residues. The wider cavity of BChE allows forming these interactions since bulky phenylalanine (Phe330) in AChE is replaced by alanine (Ala328) in BChE.
Agradecimentos
M,G acknowledge to FONDECYT project number 1200531, R,G acknowledge to scholarship ANID number 21191215, O,C acknowledge to FONDECYT project No. 3170012, and Fondequip Grants EQM170172
Referências
ARYA, A.; CHAHAL, R.; RAO, R.; RAHMAN, Md. H.; KAUSHIK, D.; AKHTAR, M. F.; SALEEM, A.; KHALIFA, S. M. A.; EL-SEEDI, H. R.; KAMEL, M.; ALBADRANI, G. M.; ABDEL-DAIM, M. M.; MITTAL, V. Acetylcholinesterase Inhibitory Potential of Various Sesquiterpene Analogues for Alzheimer’s Disease Therapy. Biomolecules, v.11 n.3, p. 350, 2021. https://doi.org/10.3390/biom11030350
BANERJEE, P.; ECKERT, A. O.; SCHREY, A. K.; PRIESSNER, R. ProTox-II: A Webserver for the Prediction of Toxicity of Chemicals. Nucleic Acids Research. v.46 n.W1 p.W257–W263 2018. https://doi.org/10.1093/nar/gky318
DAINA, A.; MICHIEJIN, O.; ZOETE, V. SwissADME: A Free Web Tool to Evaluate Pharmacokinetics, Drug-Likeness and Medicinal Chemistry Friendliness of Small Molecules. Scientific Reports. v.7 n.1, p.42717, 2017.
DARVESH, S. Butyrylcholinesterase as a Diagnostic and Therapeutic Target for Alzheimer’s Disease. Current Alzheimer Research. v.13 n.19, p 1173–1177, 2016.
DE SOUZA, L. G.; RENNO, M. N.; FIGUEROA-VILLAR, J. D. Coumarins as Cholinesterase Inhibitors: A Review. Chemico-Biological Interactions. v.254, p. 11–23, 2016. https://doi.org/10.1016/j.cbi.2016.05.001
ELLMAN, G. L.; COURTNEY, K. D.; ANDRES, V.; FEATHERSTONE, R. M. A New and Rapid Colorimetric Determination of Acetylcholinesterase Activity. Biochemical Pharmacology, v.7 n.2, p. 88-95, 1961. https://doi.org/10.1016/0006-2952(61)90145-9.
GREENWOOD, J. R.; CALKINS, D.; SULLIVAN, A. P.; SHELLEY, J. C. Towards the Comprehensive, Rapid, and Accurate Prediction of the Favorable Tautomeric States of Drug-like Molecules in Aqueous Solution. Journal of Computer-Aided Molecular Design. v.24 n.6-7, p. 591–604, 2010. https://doi.org/10.1007/s10822-010-9349-1.
GREIG, N. H.; UTSUKI, T.; YU, Q. S.; ZHU, X.; HOLLOWAY, H. W.; PERRY, T.; LEE, B.; INGRAM, D. K.; LAHIRI, D. K. A New Therapeutic Target in Alzheimer’s Disease Treatment: Attention to Butyrylcholinesterase. Current Medical Research and Opinion. v.17 n.3, p. 159–165, 2001.
KUSHWAHA, P.; FATIMA, S.; UPADHYAY, A.; GUPTA, S.; BHAGWATI, S.; BAGHEL, T.; SIDDIQI, M. I.; NAZIR, A. SASHIDHARA, K. V. Synthesis, Biological Evaluation and Molecular Dynamic Simulations of Novel Benzofuran–Tetrazole Derivatives as Potential Agents against Alzheimer’s Disease. Bioorganic & Medicinal Chemistry Letters, v.29 n.1, p. 66-72, 2019. https://doi.org/10.1016/j.bmcl.2018.11.005
LAMBRUSCHINI, C.; GALANTE, D.; MONI, L.; FERRARO, F.; GANCIA, G.; RIVA, R.; TRAVERSO, A.; BANFI, L.; D´ARRIGO, C. Multicomponent, Fragment–Based Synthesis of Polyphenol-Containing Peptidomimetics and Their Inhibiting Activity on Beta–Amyloid Oligomerization. Organic & Biomolecular Chemistry, v.15 n.44, p.9331-9351, 2017. https://doi.org/10.1039/C7OB02182H
LIPINSKI, C. A.; LOMBARDO, F.; DOMINY, B. W.; FEENEY, P. J. Experimental and Computational Approaches to Estimate Solubility and Permeability in Drug Discovery and Development Settings. Advanced Drug Delivery Reviews. v.64 n.4, p.4-17, 2012.
LIU, S.; PARK, S.; ALLINGTON, G.; PRELLI, F.; SUN, Y.; MARTA_ARIZA, M.; SCHOLZOVA, H.; BISWAS, G.; BROWN, B.; VERGHESE, P. B.; MEHTA, P. D.; KWON, Y. U.; EISNIEWSKI, T. Targeting Apolipoprotein E/Amyloid β Binding by Peptoid CPO_Aβ17–21 P Ameliorates Alzheimer’s Disease Related Pathology and Cognitive Decline. Scientific Reports, v.7 n.1, p.8009-9351, 2017, https://doi.org/10.1038/s41598-017-08604-8.
LOU, Y.; VALI, S.; SUN, S.; CHEN, X.; LIANG, X.; DROZHZHINA, T.; POPUGAEVA, E.; BEZPROZVANNY, I. Aβ42–Binding Peptoids as Amyloid Aggregation Inhibitors and Detection Ligands. ACS Chemical Neuroscience, v.4 n.6, p.952-962, 2013. https://doi.org/10.1021/cn400011f
MARCACCINI, S.; TORROBA, T. The Use of the Ugi Four–Component Condensation. Nature Protocol v.2 n.3, p. 632–639, 2007.
MARUCCI, G.; BUCCIONI, M.; BEN, D. D.; LAMBERTUCCI, C.; VOLPINI, R.; AMENTA, F. Efficacy of Acetylcholinesterase Inhibitors in Alzheimer’s Disease. Neuropharmacology. v.190, p. 108352, 2021. https://doi.org/10.1016/j.neuropharm.2020.108352
MESULAM, M.; GUILLOZET, A.; SHAW, P.; QUINN, B. Widely Spread Butyrylcholinesterase Can Hydrolyze Acetylcholine in the Normal and Alzheimer Brain. Neurobiology of Disease. v.9 n.1, p.88-93, 2002. https://doi.org/10.1006/nbdi.2001.0462.
MUSHTAQ, G.; GREIG, N.; KHAN, J.; KAMAL, M. Status of Acetylcholinesterase and Butyrylcholinesterase in Alzheimer‘s Disease and Type 2 Diabetes Mellitus. CNS & Neurological Disorders - Drug Targets, v.13 n.8, p.1432-1439, 2014. https://doi.org/10.2174/1871527313666141023141545.
PATIL, P.; AHMADIAN-MOGHADDAM, M. DÖMLING, A. Isocyanide 2.0. Green Chemistry. v.22 n.20, p. 6902–6911, 2020.
https://doi.org/10.1039/D0GC02722G
PRADHAN, K.; DAS, G.; MONDAL, P.; KHAN, J.; BARMAN, S.; GHOSH, S. Genesis of Neuroprotective Peptoid from Aβ30–34 Inhibits Aβ Aggregation and AChE Activity. ACS Chem. Neurosci. ACS Chemical Neuroscience, v.9 n.12, p.2929-2940, 2018. https://doi.org/10.1021/acschemneuro.8b00071
PRENT-PEÑALOSA, L.; F:DE LA TORRE, A.; VELAZQUEZ-LIBERA, J. L. GUTIERREZ, M.; CABALLERO, J. Synthesis of DiN–Substituted Glycyl–Phenylalanine Derivatives by Using Ugi Four Component Reaction and Their Potential as Acetylcholinesterase Inhibitors. Molecules, v.24 n.1, p.189, 2019. https://doi.org/10.3390/molecules24010189.
SONG, Q. YAN, L. ZHONGCHENG, C. HONGYAN, L. CHAOQUAN, T. ZIYI, Y. XIAOMING, Q. ZHENGHUAI, T. YONG, D Discovery of novel 2,5-dihydroxyterephthalamide derivatives as multifunctional agents for the treatment of Alzheimer’s disease. Bioorganic & Medicinal Chemistry, v.26 n.23-24, p. 6115-6127, 2018. https://doi.org/10.1016/j.bmc.2018.11.015
ZUCKERMANN, R. N. Peptoid Origins. Biopolymers, v.96 n.5, p.545-555, 2011. https://doi.org/10.1002/bip.21573